Partial List of Superconductors to Build Out
3 posters
Page 2 of 2
Page 2 of 2 • 1, 2
Re: Partial List of Superconductors to Build Out
Room Temperature Superconductivity Found in Graphite Grains
Water-soaked grains of carbon superconduct at room temperature, claim a team of physicists from Germany
September 12, 2012
Here’s an interesting recipe. Take a spoonful of graphite powder and stir it into a glass of water. Leave for 24 hours at room temperature and then filter the powder. Finally, bake overnight at 100 degrees C and allow to cool.
And voila! A material that superconducts at over 300 kelvin–room temperature. At least that’s the claim today from Pablo Esquinazi and buddies at the University of Leipzig in Germany.
If that sounds too good to be true, it’s worth taking a look at the claim in more detail since there are more than a few caveats.
First, this is not a conventional bulk material. The claim from Germany is that the superconductivity occurs at the interface between grains of graphite after they have dried out.
So that’s a surface effect which involves only a tiny fraction of the total mass of carbon in the powder–just 0.0001 per cent of the mass, according to Esquinazi and co.
What’s more the effect is clearly fragile. Esquinazi and co say the superconductivity disappears if the treated powder is pressed into pellets.
So whatever allows the superconductivity to occur at the grain interfaces is destroyed when the grains are pressed together.
Finally, the experimental evidence is tantalising rather than definitive. In ordinary circumstances, claims for superconductivity require three different lines of evidence. First, there is zero resistance. Second there is the Meissner effect in which the sample reflects an external magnetic field. And finally there must be evidence of a superconducting phase transition, such as a sudden change in the material’s magnetic properties when superconductivity occurs.
It’s this final effect that Esquinazi and co discuss in their paper. They say that the material’s magnetic properties such as its magnetic moment change in a way that is consistent with the presence of superconducting vortices.
They point out that a similar effect occurs in other granular superconducting oxides. And they also say that similar effects have been observed several times in carbon -based materials over the last 40 years or so.
These guys hypothesise that the water dopes the graphite surface with hydrogen and this plays some role in linking the grains together. This, they say, explains the superconductivity and why pressing grain breaks the bonds between them.
That’s interesting but by no means a slam dunk. However, it is likely to trigger a flurry of work investigating the properties of soaked graphite in more detail.
Esquinazi have not been able to measure the superconducting properties of individual grains. So that will be a priority in future work. Physicists will want to see evidence of zero conductivity and the Meissner effect in these particles.
After that, it will be important to work out the structure of the superconducting carbon and role that hydrogen plays (if at all).
Esquinazi and co raise the possibility that there may be some like to the increase in superconducting temperature of certain materials after they had been soaked in alcohol, a finding we discussed earlier this year.
Finally, it’s hard to imagine that superconducting grains will be of much use in the long term so physicists will want to find a way of reproducing the effect in a bulk material–along a wire, for example.
So there’s plenty of work ahead. Significantly, Esquinazi and co have waited until their work has been published in a peer reviewed journal before placing their paper on the arXiv.
That should give physicists confidence that there is something worth pursuing here. And of course, the implications and rewards are potentially huge, which adds a modicum of extra incentive.
Esquinazi and co are clearly aware of this. They finish their paper like this: “The overall results indicate that room temperature superconductivity appears to be reachable and that the here used or similar methods may pave the way for a new generation of superconducting devices with unexpected benefits for society.”
We’ll be watching.
Ref: arxiv.org/abs/1209.1938: Can Doping Graphite Trigger Room Temperature Superconductivity? Evidence For Granular High-Temperature Superconductivity in Water-Treated Graphite Powder
https://www.technologyreview.com/2012/09/12/183861/room-temperature-superconductivity-found-in-graphite-grains/
Water-soaked grains of carbon superconduct at room temperature, claim a team of physicists from Germany
September 12, 2012
Here’s an interesting recipe. Take a spoonful of graphite powder and stir it into a glass of water. Leave for 24 hours at room temperature and then filter the powder. Finally, bake overnight at 100 degrees C and allow to cool.
And voila! A material that superconducts at over 300 kelvin–room temperature. At least that’s the claim today from Pablo Esquinazi and buddies at the University of Leipzig in Germany.
If that sounds too good to be true, it’s worth taking a look at the claim in more detail since there are more than a few caveats.
First, this is not a conventional bulk material. The claim from Germany is that the superconductivity occurs at the interface between grains of graphite after they have dried out.
So that’s a surface effect which involves only a tiny fraction of the total mass of carbon in the powder–just 0.0001 per cent of the mass, according to Esquinazi and co.
What’s more the effect is clearly fragile. Esquinazi and co say the superconductivity disappears if the treated powder is pressed into pellets.
So whatever allows the superconductivity to occur at the grain interfaces is destroyed when the grains are pressed together.
Finally, the experimental evidence is tantalising rather than definitive. In ordinary circumstances, claims for superconductivity require three different lines of evidence. First, there is zero resistance. Second there is the Meissner effect in which the sample reflects an external magnetic field. And finally there must be evidence of a superconducting phase transition, such as a sudden change in the material’s magnetic properties when superconductivity occurs.
It’s this final effect that Esquinazi and co discuss in their paper. They say that the material’s magnetic properties such as its magnetic moment change in a way that is consistent with the presence of superconducting vortices.
They point out that a similar effect occurs in other granular superconducting oxides. And they also say that similar effects have been observed several times in carbon -based materials over the last 40 years or so.
These guys hypothesise that the water dopes the graphite surface with hydrogen and this plays some role in linking the grains together. This, they say, explains the superconductivity and why pressing grain breaks the bonds between them.
That’s interesting but by no means a slam dunk. However, it is likely to trigger a flurry of work investigating the properties of soaked graphite in more detail.
Esquinazi have not been able to measure the superconducting properties of individual grains. So that will be a priority in future work. Physicists will want to see evidence of zero conductivity and the Meissner effect in these particles.
After that, it will be important to work out the structure of the superconducting carbon and role that hydrogen plays (if at all).
Esquinazi and co raise the possibility that there may be some like to the increase in superconducting temperature of certain materials after they had been soaked in alcohol, a finding we discussed earlier this year.
Finally, it’s hard to imagine that superconducting grains will be of much use in the long term so physicists will want to find a way of reproducing the effect in a bulk material–along a wire, for example.
So there’s plenty of work ahead. Significantly, Esquinazi and co have waited until their work has been published in a peer reviewed journal before placing their paper on the arXiv.
That should give physicists confidence that there is something worth pursuing here. And of course, the implications and rewards are potentially huge, which adds a modicum of extra incentive.
Esquinazi and co are clearly aware of this. They finish their paper like this: “The overall results indicate that room temperature superconductivity appears to be reachable and that the here used or similar methods may pave the way for a new generation of superconducting devices with unexpected benefits for society.”
We’ll be watching.
Ref: arxiv.org/abs/1209.1938: Can Doping Graphite Trigger Room Temperature Superconductivity? Evidence For Granular High-Temperature Superconductivity in Water-Treated Graphite Powder
https://www.technologyreview.com/2012/09/12/183861/room-temperature-superconductivity-found-in-graphite-grains/
Chromium6- Posts : 729
Join date : 2019-11-29
Re: Partial List of Superconductors to Build Out
When hydrogen is incorporated into the nickelate structure, it is not a superconductor. Credit: TU Wien Nickelate
Last summer, a new age for high-temperature superconductivity was proclaimed—the nickel age. It was discovered that there are promising superconductors in a special class of materials, the so-called nickelates, which can conduct electric current without any resistance even at high temperatures.
However, it soon became apparent that these initially spectacular results from Stanford could not be reproduced by other research groups. TU Wien (Vienna) has now found the reason for this: In some nickelates additional hydrogen atoms are incorporated into the material structure. This completely changes the electrical behaviour of the material. In the production of the new superconductors, this effect must now be taken into account.
The search for High-Temperature Superconductors
Some materials are only superconducting near absolute temperature zero—such superconductors are not suitable for technical applications. Therefore, for decades, people have been looking for materials that remain superconducting even at higher temperatures. In the 1980s, "high-temperature superconductors" were discovered. What is referred to as "high temperatures" in this context, however, is still very cold: even high-temperature superconductors must be cooled strongly in order to obtain their superconducting properties. Therefore, the search for new superconductors at even higher temperatures continues.
"For a long time, special attention was paid to so-called cuprates, i.e. compounds containing copper. This is why we also speak of the copper age", explains Prof. Karsten Held from the Institute of Solid State Physics at TU Wien. "With these cuprates, some important progress was made, even though there are still many open questions in the theory of high-temperature superconductivity today".
But for some time now, other possibilities have also been under consideration. There was already a so-called "iron age" based on iron-containing superconductors. In summer 2019, the research group of Harold Y. Hwang's research group from Stanford then succeeded in demonstrating high-temperature superconductivity in nickelates. "Based on our calculations, we already proposed nickelates as superconductors 10 years ago, but they were somewhat different from the ones that have now been discovered. They are related to cuprates, but contain nickel instead of copper atoms," says Karsten Held.
The Trouble with Hydrogen
After some initial enthusiasm, however, it has become apparent in recent months that nickelate superconductors are more difficult to produce than initially thought. Other research groups reported that their nickelates do not have superconducting properties. This apparent contradiction has now been clarified at TU Wien.
"We analysed the nickelates with the help of supercomputers and found that they are extremely receptive to hydrogen into the material," reports Liang Si (TU Vienna). In the synthesis of certain nickelates, hydrogen atoms can be incorporated, which completely changes the electronic properties of the material. "However, this does not happen with all nickelates," says Liang Si, "Our calculations show that for most of them, it is energetically more favourable to incorporate hydrogen, but not for the nickelates from Stanford. Even small changes in the synthesis conditions can make a difference." Last Friday the group around Ariando Ariando from the NUS Singapore could report that they also succeeded in producing superconducting nickelates. They let the hydrogen that is released in the production process escape immediately.
Calculating the Critical Temperature with Supercomputers
At TU Wien new computer calculation methods are being developed and used to understand and predict the properties of nickelates. "Since a large number of quantum-physical particles always play a role here at the same time, the calculations are extremely complex," says Liang Si, "But by combining different methods, we are now even able to estimate the critical temperature up to which the various materials are superconducting. Such reliable calculations have not been possible before."In particular, the team at TU Wien was able to calculate the allowed range of strontium concentration for which the nickelates are superconducting—and this prediction has now been confirmed in experiment.
More at link: https://phys.org/news/2020-04-superconductivity-hydrogen-fault.html
----------
March 13, 2020
Modified nickelate materials could improve understanding of high-temperature superconductivity
by RIKEN
https://phys.org/news/2020-03-nickelate-materials-high-temperature-superconductivity.html
....
Conventional superconductivity depends on a form of electron pairing that occurs only at extremely low temperatures, so superconducting devices must be cooled with expensive liquefied gases. But about 30 years ago, researchers discovered that some cuprate materials could become superconductors at relatively warm temperatures, up to −140 degrees Celsius. The underlying cause of this high-temperature superconductivity is still not understood.
In 2019, researchers found that a strontium-doped neodymium nickel oxide (Nd0.8Sr0.2NiO2) could superconduct below −258 degrees Celsius. The discovery attracted attention not because of the temperature, but because this nickelate material has a very similar crystal structure to the cuprates, and may serve as a test-bed to better understand how superconductivity works in these materials.
The nickelate material consists of alternating layers of Nd and NiO2. Yusuke Nomura at the RIKEN Center for Emergent Matter Science and colleagues have now studied how the interactions between certain electrons in these two layers might influence superconductivity.
The team's calculations showed that the electrons in the NiO2 layer strongly interact with each other, which is similar to cuprates where the strong correlation in the CuO2 layer is believed to play a key role in their high-temperature superconductivity. However, there is a difference between the nickelates and the cuprates: in the nickelates, electrons in the neodymium layer are partially occupied and form the Fermi pocket, a relatively small region in the Brillouin zone surrounded by the Fermi surface. These pockets do not appear in cuprates, which may make the nickelate material an imperfect analogue for cuprates.
Nomura's team used computational models to study whether the pockets could be eliminated by tweaking the chemical composition of the material and hence create a nickelate that is a better match for the cuprates. They found that two compounds could fit the bill: sodium neodymium nickel oxide (NaNd2NiO4) and sodium calcium nickel oxide (NaCa2NiO3). "If the proposed nickelates are synthesized, they will be real nickel analogues of cuprate superconductors," Nomura notes.
"The next stage is to clarify the difference and similarity between the nickelates and cuprates in a more systematic way, and get deeper insight into the superconducting mechanism in both systems," he adds.
..............
NaNd2NiO4

NaCa2NiO3

NiO2

Last summer, a new age for high-temperature superconductivity was proclaimed—the nickel age. It was discovered that there are promising superconductors in a special class of materials, the so-called nickelates, which can conduct electric current without any resistance even at high temperatures.
However, it soon became apparent that these initially spectacular results from Stanford could not be reproduced by other research groups. TU Wien (Vienna) has now found the reason for this: In some nickelates additional hydrogen atoms are incorporated into the material structure. This completely changes the electrical behaviour of the material. In the production of the new superconductors, this effect must now be taken into account.
The search for High-Temperature Superconductors
Some materials are only superconducting near absolute temperature zero—such superconductors are not suitable for technical applications. Therefore, for decades, people have been looking for materials that remain superconducting even at higher temperatures. In the 1980s, "high-temperature superconductors" were discovered. What is referred to as "high temperatures" in this context, however, is still very cold: even high-temperature superconductors must be cooled strongly in order to obtain their superconducting properties. Therefore, the search for new superconductors at even higher temperatures continues.
"For a long time, special attention was paid to so-called cuprates, i.e. compounds containing copper. This is why we also speak of the copper age", explains Prof. Karsten Held from the Institute of Solid State Physics at TU Wien. "With these cuprates, some important progress was made, even though there are still many open questions in the theory of high-temperature superconductivity today".
But for some time now, other possibilities have also been under consideration. There was already a so-called "iron age" based on iron-containing superconductors. In summer 2019, the research group of Harold Y. Hwang's research group from Stanford then succeeded in demonstrating high-temperature superconductivity in nickelates. "Based on our calculations, we already proposed nickelates as superconductors 10 years ago, but they were somewhat different from the ones that have now been discovered. They are related to cuprates, but contain nickel instead of copper atoms," says Karsten Held.
The Trouble with Hydrogen
After some initial enthusiasm, however, it has become apparent in recent months that nickelate superconductors are more difficult to produce than initially thought. Other research groups reported that their nickelates do not have superconducting properties. This apparent contradiction has now been clarified at TU Wien.
"We analysed the nickelates with the help of supercomputers and found that they are extremely receptive to hydrogen into the material," reports Liang Si (TU Vienna). In the synthesis of certain nickelates, hydrogen atoms can be incorporated, which completely changes the electronic properties of the material. "However, this does not happen with all nickelates," says Liang Si, "Our calculations show that for most of them, it is energetically more favourable to incorporate hydrogen, but not for the nickelates from Stanford. Even small changes in the synthesis conditions can make a difference." Last Friday the group around Ariando Ariando from the NUS Singapore could report that they also succeeded in producing superconducting nickelates. They let the hydrogen that is released in the production process escape immediately.
Calculating the Critical Temperature with Supercomputers
At TU Wien new computer calculation methods are being developed and used to understand and predict the properties of nickelates. "Since a large number of quantum-physical particles always play a role here at the same time, the calculations are extremely complex," says Liang Si, "But by combining different methods, we are now even able to estimate the critical temperature up to which the various materials are superconducting. Such reliable calculations have not been possible before."In particular, the team at TU Wien was able to calculate the allowed range of strontium concentration for which the nickelates are superconducting—and this prediction has now been confirmed in experiment.
More at link: https://phys.org/news/2020-04-superconductivity-hydrogen-fault.html
----------
March 13, 2020
Modified nickelate materials could improve understanding of high-temperature superconductivity
by RIKEN
https://phys.org/news/2020-03-nickelate-materials-high-temperature-superconductivity.html
....
Conventional superconductivity depends on a form of electron pairing that occurs only at extremely low temperatures, so superconducting devices must be cooled with expensive liquefied gases. But about 30 years ago, researchers discovered that some cuprate materials could become superconductors at relatively warm temperatures, up to −140 degrees Celsius. The underlying cause of this high-temperature superconductivity is still not understood.
In 2019, researchers found that a strontium-doped neodymium nickel oxide (Nd0.8Sr0.2NiO2) could superconduct below −258 degrees Celsius. The discovery attracted attention not because of the temperature, but because this nickelate material has a very similar crystal structure to the cuprates, and may serve as a test-bed to better understand how superconductivity works in these materials.
The nickelate material consists of alternating layers of Nd and NiO2. Yusuke Nomura at the RIKEN Center for Emergent Matter Science and colleagues have now studied how the interactions between certain electrons in these two layers might influence superconductivity.
The team's calculations showed that the electrons in the NiO2 layer strongly interact with each other, which is similar to cuprates where the strong correlation in the CuO2 layer is believed to play a key role in their high-temperature superconductivity. However, there is a difference between the nickelates and the cuprates: in the nickelates, electrons in the neodymium layer are partially occupied and form the Fermi pocket, a relatively small region in the Brillouin zone surrounded by the Fermi surface. These pockets do not appear in cuprates, which may make the nickelate material an imperfect analogue for cuprates.
Nomura's team used computational models to study whether the pockets could be eliminated by tweaking the chemical composition of the material and hence create a nickelate that is a better match for the cuprates. They found that two compounds could fit the bill: sodium neodymium nickel oxide (NaNd2NiO4) and sodium calcium nickel oxide (NaCa2NiO3). "If the proposed nickelates are synthesized, they will be real nickel analogues of cuprate superconductors," Nomura notes.
"The next stage is to clarify the difference and similarity between the nickelates and cuprates in a more systematic way, and get deeper insight into the superconducting mechanism in both systems," he adds.
..............
NaNd2NiO4

NaCa2NiO3

NiO2

Chromium6- Posts : 729
Join date : 2019-11-29
Re: Partial List of Superconductors to Build Out
The Dr. probably spilled his Saki at a lab party...
---------
Why the Change to a Superconductor when Simmered in Alcoholic Beverages?
New Possibility in Research and Development of Superconductors by Elucidation of Causative Agent and Mechanism
2012.07.16
(2012.09.12 Update)
National Institute for Materials Science
Institute for Advanced Biosciences, Keio University
In previous research, the National Institute for Materials Science discovered that iron telluride compounds [Fe(Te,S) system], which are iron-based superconducting related substances, become superconductors when simmered in alcoholic beverages.In the research reported here, NIMS and the Institute for Advanced Biosciences of Keio University identified substances in alcoholic beverages which induce superconductivity and clarified the mechanism of why they do so.
概要
In previous research, the National Institute for Materials Science (NIMS, Tsukuba City, Ibaraki Prefecture; President: Sukekatsu Ushioda) discovered that iron telluride compounds [Fe(Te,S) system], which are iron-based superconducting related substances, become superconductors when simmered in alcoholic beverages. (This discovery was announced in a joint press release by NIMS and the Japan Science and Technology Agency (JST) dated July 27, 2010.) In the research reported here, NIMS and the Institute for Advanced Biosciences (IAB, Tsuruoka City, Yamagata Prefecture; Director: Masaru Tomita) of Keio University identified substances in alcoholic beverages which induce superconductivity and clarified the mechanism of why they do so.
Using a metabolomics technique developed by IAB, which is called Capillary Electrophoresis Time-of-Flight Mass Spectrometer (CE-TOFMS), comprehensive quantification was performed for the components contained in 6 types of alcoholic beverages (red wine, white wine, beer, whisky, Japanese sake, and shochu
( a type of Japanese distilled alcoholic beverage)), and the possible candidate substances which induce superconductivity were narrowed down by comparing those components and the volume fractions of superconducting phases.
It was found that malic acid, citric acid, and β-alanine, which have particularly high correlations among the candidate substances, actually had an influence in inducing superconductivity.
Focusing on the fact that all the candidate substances have a chelating effect, after iron telluride sample was simmered in the alcoholic beverages and the above-mentioned 3 substances, the solutions were investigated and iron ions which appeared to have been eluted from the specimens were detected.
Based on the findings outlined above, the researchers concluded that the substances in alcoholic beverages which are responsible for inducing superconductivity are organic acids that have a chelating effect, and superconductivity is induced when these substances remove surplus iron, which suppresses superconductivity, from the specimens.
The negative effect of surplus iron on superconductivity can also occur to a significant extent in other iron-based superconductors, these research results are expected to provide new guidelines for research and development of iron-based superconductors.
This research was the result of joint research by a team headed by Dr. Yoshihiko Takano, Group Leader of the Nano Frontier Materials Group, and a team led by Dr. Dan Sato, Project Assistant Professor of the IAB. These results are scheduled for publication in July in the Special Issue on Iron-based Superconductors of the interdisciplinary scientific journal Superconductor Science and Technology.
https://www.nims.go.jp/eng/news/press/2012/07/p201207130.html

---------
Why the Change to a Superconductor when Simmered in Alcoholic Beverages?
New Possibility in Research and Development of Superconductors by Elucidation of Causative Agent and Mechanism
2012.07.16
(2012.09.12 Update)
National Institute for Materials Science
Institute for Advanced Biosciences, Keio University
In previous research, the National Institute for Materials Science discovered that iron telluride compounds [Fe(Te,S) system], which are iron-based superconducting related substances, become superconductors when simmered in alcoholic beverages.In the research reported here, NIMS and the Institute for Advanced Biosciences of Keio University identified substances in alcoholic beverages which induce superconductivity and clarified the mechanism of why they do so.
概要
In previous research, the National Institute for Materials Science (NIMS, Tsukuba City, Ibaraki Prefecture; President: Sukekatsu Ushioda) discovered that iron telluride compounds [Fe(Te,S) system], which are iron-based superconducting related substances, become superconductors when simmered in alcoholic beverages. (This discovery was announced in a joint press release by NIMS and the Japan Science and Technology Agency (JST) dated July 27, 2010.) In the research reported here, NIMS and the Institute for Advanced Biosciences (IAB, Tsuruoka City, Yamagata Prefecture; Director: Masaru Tomita) of Keio University identified substances in alcoholic beverages which induce superconductivity and clarified the mechanism of why they do so.
Using a metabolomics technique developed by IAB, which is called Capillary Electrophoresis Time-of-Flight Mass Spectrometer (CE-TOFMS), comprehensive quantification was performed for the components contained in 6 types of alcoholic beverages (red wine, white wine, beer, whisky, Japanese sake, and shochu

It was found that malic acid, citric acid, and β-alanine, which have particularly high correlations among the candidate substances, actually had an influence in inducing superconductivity.
Focusing on the fact that all the candidate substances have a chelating effect, after iron telluride sample was simmered in the alcoholic beverages and the above-mentioned 3 substances, the solutions were investigated and iron ions which appeared to have been eluted from the specimens were detected.
Based on the findings outlined above, the researchers concluded that the substances in alcoholic beverages which are responsible for inducing superconductivity are organic acids that have a chelating effect, and superconductivity is induced when these substances remove surplus iron, which suppresses superconductivity, from the specimens.
The negative effect of surplus iron on superconductivity can also occur to a significant extent in other iron-based superconductors, these research results are expected to provide new guidelines for research and development of iron-based superconductors.
This research was the result of joint research by a team headed by Dr. Yoshihiko Takano, Group Leader of the Nano Frontier Materials Group, and a team led by Dr. Dan Sato, Project Assistant Professor of the IAB. These results are scheduled for publication in July in the Special Issue on Iron-based Superconductors of the interdisciplinary scientific journal Superconductor Science and Technology.
https://www.nims.go.jp/eng/news/press/2012/07/p201207130.html
Chromium6- Posts : 729
Join date : 2019-11-29
Re: Partial List of Superconductors to Build Out
Graphene Superconductors May Be Less Exotic Than Physicists Hoped
CONDENSED MATTER PHYSICS
Graphene Superconductors May Be Less Exotic Than Physicists Hoped
By
CHARLIE WOOD
June 14, 2021
Superconductivity has been discovered in graphene devices without any twists, suggesting the form of superconductivity in the material might be mundane after all.
An illustration showing three stacked sheets of carbon atoms, each offset from the one below by half a lattice spacing.]
ABC trilayer graphene, with layers that are shifted rather than twisted, showed flickers of superconductivity when cooled to near absolute zero.
Samuel Velasco/Quanta Magazine
Three years ago, physicists discovered that two stacked sheets of carbon with a tiny, 1.1-degree twist between them could exhibit a dazzling array of behaviors. Most famously, when cooled to low temperatures, the material conducts electricity with zero resistance.
Researchers raced to figure out why twisted bilayer graphene (as it’s called) becomes a superconductor, with a form of superconductivity that seems unusually robust. Many theorists hoped the discovery would rewrite their understanding of superconductivity, and perhaps even allow researchers to engineer materials capable of sustaining the phenomenon at higher temperatures.
But the intense focus on that twist between the graphene sheets may have been a case of misdirection. A team of physicists announced today at an online conference that they’ve observed superconductivity in a triple-decker stack of graphene with no twists at all. The discovery, led by Andrea Young and Haoxin Zhou of the University of California, Santa Barbara, could reset discussions about superconductivity in graphene. It has led some theorists to suspect that graphene’s superconductivity is the vanilla variety after all.
“That’s a very important discovery showing that superconductivity [in graphene] is, in some sense, regular,” said Sankar Das Sarma, a theoretical condensed matter physicist at the University of Maryland who was not involved in the
But the evidence for conventional superconductivity is not conclusive. And researchers note that twisted graphene’s superconductivity could still be exotic even if untwisted graphene’s isn’t.
Albert Einstein, Richard Feynman and Werner Heisenberg are just a few of the titans of 20th-century physics who tried and failed to understand why many metals carry current without resistance at low temperatures. In 1957, nearly half a century after this standard kind of superconductivity was discovered, John Bardeen, Leon Cooper and John Robert Schrieffer finally explained the phenomenon, an achievement that earned them the Nobel Prize in Physics.
They determined that sound waves in metals — ripples where atoms bunch together, called phonons — create concentrations of positive charge that attract electrons, which are negatively charged. The phonons stick electrons together into “Cooper pairs.” Coupled off in this way, electrons play by different quantum mechanical rules, fusing into a quantum fluid whose flow is no longer gummed up by the atoms in the lattice. This phonon-mediated theory, known (after its authors’ initials) as BCS, matches almost all superconductivity experiments.
Alternative ways of gluing electrons together work on paper, and experimentalists have seen signs of puzzlingly strong “unconventional” glues in some superconductors, but such claims remain unsettled.
“It’s like if someone tells you in some very distant village on some island there are people with three heads,” Das Sarma said. “You should be very, very skeptical.”
In 2018, some researchers thought they might have stumbled upon just such a mythical island of exotic superconductivity, since twisted bilayer graphene appeared to somehow bind electrons much more tightly together than most superconductors do. Excitement rose earlier this year with the discovery of superconductivity in a similar system: three layers of graphene twisted at their own special angle. Both systems shared a rare, 180-degree rotational symmetry, which theorists argued could support an especially exotic form of superconductivity based on electron vortices known as skyrmions.
But the new incarnation of superconducting graphene appears strikingly plain.
ABC trilayer graphene, as Young and his colleagues call their graphene stack, is one of the cleanest and simplest materials they could make. The second and third layers are shifted rather than twisted, each nudged over by an additional half-honeycomb, so carbon atoms below fall in the center of lattices above.
Materials are complicated, and they have a way of lying to us.
Steve Kivelson
Stacking graphene sheets is hard, with or without twists. Twisted devices are riddled with wrinkles that disrupt the magic angle in different zones, making each apparatus unique. Even when Young and colleagues manufactured their ABC trilayer devices, most attempts snapped back into an alternative stacking pattern. But — unlike the fussy twisted samples — the ones that stayed put were identical down to the last atom. The atoms “lock into place like Legos,” Young said.
Once the team had their first ABC device, they used an adjustable electric field to shuffle electrons between the pristine layers. As they tuned the electron distribution at cryogenic temperatures, they saw that the system behaved much as twisted graphene does, jumping between various types of magnetic behavior, as indicated by shifts in how the device slowed electric current. They posted their results in an April preprint.
When they examined the transitions in more detail, they identified brief flickers of zero electrical resistance — superconductivity — when the material was about one-tenth of a degree above absolute zero.
Although Young and his colleagues have no way to peep at the Cooper pairs of electrons directly, they found behavior that Bardeen, Cooper and Schrieffer would recognize: Moving electrons between the three layers increased the number of possible configurations the electrons could choose from, a quantity known as the system’s “density of states.” At high densities of states, electrons can more easily fraternize among themselves. BCS theory predicts that this electronic liberty aids the formation of Cooper pairs, and that’s what the researchers found: As the density of states rose, the material displayed two blips of superconductivity.
Since the BCS equation appears to hold, ordinary phonons might be responsible for the superconductivity.
“It’s quacking like a duck and walking like a duck,” Das Sarma said. “Phonons are natural to assume.”
Others are less convinced, noting that the evidence supporting phonons in ABC trilayer graphene remains rough. Superconductivity appears to track with the higher density of states, but that doesn’t mean the BCS equation is obeyed in detail, said Mike Zaletel, a condensed matter physicist at the University of California, Berkeley who consulted with Young during the research and helped develop the skyrmion theory of superconductivity.
In Young’s data, Zaletel sees hints of a mildly exotic sort of superconductivity — something like an island with a six-fingered population, rather than people with three heads. He explained that both flashes of superconductivity appeared immediately before the electrons organized into ferromagnetic states, where their spin directions became aligned. As regions of electrons started lining up, these fluctuating pockets of uniformity could have shepherded electrons into Cooper pairs much as phonons do.
Young’s group is already testing whether ferromagnetism is key to the onset of superconductivity in ABC trilayer graphene, or if it’s irrelevant — which would hint at conventional phonons.
With a Simple Twist, a ‘Magic’ Material Is Now the Big Thing in Physics
Room-Temperature Superconductivity Achieved for the First Time
The Quantum Secret to Superconductivity
Many physicists feel optimistic that Young’s new platform will help them figure out how electrons superconduct in graphene. The idiosyncrasies of each twisted graphene device made it impossible for even an individual lab to identically replicate its own results. ABC trilayer graphene, with its perfect layout, overcomes that challenge.
“Materials are complicated, and they have a way of lying to us,” said Steven Kivelson, a theoretical physicist at Stanford University. “What’s exciting about this development” is that it promises reproducible materials, “so that everybody can get the same answer.”
more at link: https://www.quantamagazine.org/graphene-superconductors-may-be-less-exotic-than-physicists-hoped-20210614/
CONDENSED MATTER PHYSICS
Graphene Superconductors May Be Less Exotic Than Physicists Hoped
By
CHARLIE WOOD
June 14, 2021
Superconductivity has been discovered in graphene devices without any twists, suggesting the form of superconductivity in the material might be mundane after all.
An illustration showing three stacked sheets of carbon atoms, each offset from the one below by half a lattice spacing.]
ABC trilayer graphene, with layers that are shifted rather than twisted, showed flickers of superconductivity when cooled to near absolute zero.
Samuel Velasco/Quanta Magazine
Three years ago, physicists discovered that two stacked sheets of carbon with a tiny, 1.1-degree twist between them could exhibit a dazzling array of behaviors. Most famously, when cooled to low temperatures, the material conducts electricity with zero resistance.
Researchers raced to figure out why twisted bilayer graphene (as it’s called) becomes a superconductor, with a form of superconductivity that seems unusually robust. Many theorists hoped the discovery would rewrite their understanding of superconductivity, and perhaps even allow researchers to engineer materials capable of sustaining the phenomenon at higher temperatures.
But the intense focus on that twist between the graphene sheets may have been a case of misdirection. A team of physicists announced today at an online conference that they’ve observed superconductivity in a triple-decker stack of graphene with no twists at all. The discovery, led by Andrea Young and Haoxin Zhou of the University of California, Santa Barbara, could reset discussions about superconductivity in graphene. It has led some theorists to suspect that graphene’s superconductivity is the vanilla variety after all.
“That’s a very important discovery showing that superconductivity [in graphene] is, in some sense, regular,” said Sankar Das Sarma, a theoretical condensed matter physicist at the University of Maryland who was not involved in the
But the evidence for conventional superconductivity is not conclusive. And researchers note that twisted graphene’s superconductivity could still be exotic even if untwisted graphene’s isn’t.
Albert Einstein, Richard Feynman and Werner Heisenberg are just a few of the titans of 20th-century physics who tried and failed to understand why many metals carry current without resistance at low temperatures. In 1957, nearly half a century after this standard kind of superconductivity was discovered, John Bardeen, Leon Cooper and John Robert Schrieffer finally explained the phenomenon, an achievement that earned them the Nobel Prize in Physics.
They determined that sound waves in metals — ripples where atoms bunch together, called phonons — create concentrations of positive charge that attract electrons, which are negatively charged. The phonons stick electrons together into “Cooper pairs.” Coupled off in this way, electrons play by different quantum mechanical rules, fusing into a quantum fluid whose flow is no longer gummed up by the atoms in the lattice. This phonon-mediated theory, known (after its authors’ initials) as BCS, matches almost all superconductivity experiments.
Alternative ways of gluing electrons together work on paper, and experimentalists have seen signs of puzzlingly strong “unconventional” glues in some superconductors, but such claims remain unsettled.
“It’s like if someone tells you in some very distant village on some island there are people with three heads,” Das Sarma said. “You should be very, very skeptical.”
In 2018, some researchers thought they might have stumbled upon just such a mythical island of exotic superconductivity, since twisted bilayer graphene appeared to somehow bind electrons much more tightly together than most superconductors do. Excitement rose earlier this year with the discovery of superconductivity in a similar system: three layers of graphene twisted at their own special angle. Both systems shared a rare, 180-degree rotational symmetry, which theorists argued could support an especially exotic form of superconductivity based on electron vortices known as skyrmions.
But the new incarnation of superconducting graphene appears strikingly plain.
ABC trilayer graphene, as Young and his colleagues call their graphene stack, is one of the cleanest and simplest materials they could make. The second and third layers are shifted rather than twisted, each nudged over by an additional half-honeycomb, so carbon atoms below fall in the center of lattices above.
Materials are complicated, and they have a way of lying to us.

Steve Kivelson
Stacking graphene sheets is hard, with or without twists. Twisted devices are riddled with wrinkles that disrupt the magic angle in different zones, making each apparatus unique. Even when Young and colleagues manufactured their ABC trilayer devices, most attempts snapped back into an alternative stacking pattern. But — unlike the fussy twisted samples — the ones that stayed put were identical down to the last atom. The atoms “lock into place like Legos,” Young said.
Once the team had their first ABC device, they used an adjustable electric field to shuffle electrons between the pristine layers. As they tuned the electron distribution at cryogenic temperatures, they saw that the system behaved much as twisted graphene does, jumping between various types of magnetic behavior, as indicated by shifts in how the device slowed electric current. They posted their results in an April preprint.
When they examined the transitions in more detail, they identified brief flickers of zero electrical resistance — superconductivity — when the material was about one-tenth of a degree above absolute zero.
Although Young and his colleagues have no way to peep at the Cooper pairs of electrons directly, they found behavior that Bardeen, Cooper and Schrieffer would recognize: Moving electrons between the three layers increased the number of possible configurations the electrons could choose from, a quantity known as the system’s “density of states.” At high densities of states, electrons can more easily fraternize among themselves. BCS theory predicts that this electronic liberty aids the formation of Cooper pairs, and that’s what the researchers found: As the density of states rose, the material displayed two blips of superconductivity.
Since the BCS equation appears to hold, ordinary phonons might be responsible for the superconductivity.
“It’s quacking like a duck and walking like a duck,” Das Sarma said. “Phonons are natural to assume.”
Others are less convinced, noting that the evidence supporting phonons in ABC trilayer graphene remains rough. Superconductivity appears to track with the higher density of states, but that doesn’t mean the BCS equation is obeyed in detail, said Mike Zaletel, a condensed matter physicist at the University of California, Berkeley who consulted with Young during the research and helped develop the skyrmion theory of superconductivity.
In Young’s data, Zaletel sees hints of a mildly exotic sort of superconductivity — something like an island with a six-fingered population, rather than people with three heads. He explained that both flashes of superconductivity appeared immediately before the electrons organized into ferromagnetic states, where their spin directions became aligned. As regions of electrons started lining up, these fluctuating pockets of uniformity could have shepherded electrons into Cooper pairs much as phonons do.
Young’s group is already testing whether ferromagnetism is key to the onset of superconductivity in ABC trilayer graphene, or if it’s irrelevant — which would hint at conventional phonons.
With a Simple Twist, a ‘Magic’ Material Is Now the Big Thing in Physics
Room-Temperature Superconductivity Achieved for the First Time
The Quantum Secret to Superconductivity
Many physicists feel optimistic that Young’s new platform will help them figure out how electrons superconduct in graphene. The idiosyncrasies of each twisted graphene device made it impossible for even an individual lab to identically replicate its own results. ABC trilayer graphene, with its perfect layout, overcomes that challenge.
“Materials are complicated, and they have a way of lying to us,” said Steven Kivelson, a theoretical physicist at Stanford University. “What’s exciting about this development” is that it promises reproducible materials, “so that everybody can get the same answer.”
more at link: https://www.quantamagazine.org/graphene-superconductors-may-be-less-exotic-than-physicists-hoped-20210614/
Chromium6- Posts : 729
Join date : 2019-11-29
Re: Partial List of Superconductors to Build Out
Physicists discover a “family” of robust, superconducting graphene structures
The findings could inform the design of practical superconducting devices.
Jennifer Chu | MIT News Office
Publication Date:July 8, 2022
(more at link)
When it comes to graphene, it appears that superconductivity runs in the family.
Graphene is a single-atom-thin material that can be exfoliated from the same graphite that is found in pencil lead. The ultrathin material is made entirely from carbon atoms that are arranged in a simple hexagonal pattern, similar to that of chicken wire. Since its isolation in 2004, graphene has been found to embody numerous remarkable properties in its single-layer form.
In 2018, MIT researchers found that if two graphene layers are stacked at a very specific “magic” angle, the twisted bilayer structure could exhibit robust superconductivity, a widely sought material state in which an electrical current can flow through with zero energy loss. Recently, the same group found a similar superconductive state exists in twisted trilayer graphene — a structure made from three graphene layers stacked at a precise, new magic angle.
Now the team reports that — you guessed it — four and five graphene layers can be twisted and stacked at new magic angles to elicit robust superconductivity at low temperatures. This latest discovery, published this week in Nature Materials, establishes the various twisted and stacked configurations of graphene as the first known “family” of multilayer magic-angle superconductors. The team also identified similarities and differences between graphene family members.
The findings could serve as a blueprint for designing practical, room-temperature superconductors. If the properties among family members could be replicated in other, naturally conductive materials, they could be harnessed, for instance, to deliver electricity without dissipation or build magnetically levitating trains that run without friction.
“The magic-angle graphene system is now a legitimate ‘family,’ beyond a couple of systems,” says lead author Jeong Min (Jane) Park, a graduate student in MIT’s Department of Physics. “Having this family is particularly meaningful because it provides a way to design robust superconductors.”
Park’s MIT co-authors include Yuan Cao, Li-Qiao Xia, Shuwen Sun, and Pablo Jarillo-Herrero, the Cecil and Ida Green Professor of Physics, along with Kenji Watanabe and Takashi Taniguchi of the National Institute for Materials Science in Tsukuba, Japan.
“No limit”
Jarillo-Herrero’s group was the first to discover magic-angle graphene, in the form of a bilayer structure of two graphene sheets placed one atop the other and slightly offset at a precise angle of 1.1 degrees. This twisted configuration, known as a moiré superlattice, transformed the material into a strong and persistent superconductor at ultralow temperatures.
The researchers also found that the material exhibited a type of electronic structure known as a “flat band,” in which the material’s electrons have the same energy, regardless of their momentum. In this flat band state, and at ultracold temperatures, the normally frenetic electrons collectively slow down enough to pair up in what are known as Cooper pairs — essential ingredients of superconductivity that can flow through the material without resistance.
While the researchers observed that twisted bilayer graphene exhibited both superconductivity and a flat band structure, it wasn’t clear whether the former arose from the latter.
“There was no proof a flat band structure led to superconductivity,” Park says. “Other groups since then have produced other twisted structures from other materials that have some flattish band, but they didn’t really have robust superconductivity. So we wondered: Could we produce another flat band superconducting device?”
As they considered this question, a group from Harvard University derived calculations that confirmed mathematically that three graphene layers, twisted at 1.6 degrees, would exhibit also flat bands, and suggested they may superconduct. They went on to show there should be no limit to the number of graphene layers that exhibit superconductivity, if stacked and twisted in just the right way, at angles they also predicted. Finally, they proved they could mathematically relate every multilayer structure to a common flat band structure — strong proof that a flat band may lead to robust superconductivity.
“They worked out there may be this entire hierarchy of graphene structures, to infinite layers, that might correspond to a similar mathematical expression for a flat band structure,” Park says.
Shortly after that work, Jarillo-Herrero’s group found that, indeed, superconductivity and a flat band emerged in twisted trilayer graphene — three graphene sheets, stacked like a cheese sandwich, the middle cheese layer shifted by 1.6 degrees with respect to the sandwiched outer layers. But the trilayer structure also showed subtle differences compared to its bilayer counterpart.
“That made us ask, where do these two structures fit in terms of the whole class of materials, and are they from the same family?” Park says.
An unconventional family
In the current study, the team looked to level up the number of graphene layers. They fabricated two new structures, made from four and five graphene layers, respectively. Each structure is stacked alternately, similar to the shifted cheese sandwich of twisted trilayer graphene.
The team kept the structures in a refrigerator below 1 kelvin (about -273 degrees Celsius), ran electrical current through each structure, and measured the output under various conditions, similar to tests for their bilayer and trilayer systems.
Overall, they found that both four- and five-layer twisted graphene also exhibit robust superconductivity and a flat band. The structures also shared other similarities with their three-layer counterpart, such as their response under a magnetic field of varying strength, angle, and orientation.
These experiments showed that twisted graphene structures could be considered a new family, or class of common superconducting materials. The experiments also suggested there may be a black sheep in the family: The original twisted bilayer structure, while sharing key properties, also showed subtle differences from its siblings. For instance, the group’s previous experiments showed the structure’s superconductivity broke down under lower magnetic fields and was more uneven as the field rotated, compared to its multilayer siblings.
The team carried out simulations of each structure type, seeking an explanation for the differences between family members. They concluded that the fact that twisted bilayer graphene’s superconductivity dies out under certain magnetic conditions is simply because all of its physical layers exist in a “nonmirrored” form within the structure. In other words, there are no two layers in the structure that are mirror opposites of each other, whereas graphene’s multilayer siblings exhibit some sort of mirror symmetry. These findings suggest that the mechanism driving electrons to flow in a robust superconductive state is the same across the twisted graphene family.
“That’s quite important,” Park notes. “Without knowing this, people might think bilayer graphene is more conventional compared to multilayer structures. But we show that this entire family may be unconventional, robust superconductors.”
This research was supported, in part, by the U.S. Department of Energy, the National Science Foundation, the Air Force Office of Scientific Research, the Gordon and Betty Moore Fundation, the Ramon Areces Foundation, and the CIFAR Program on Quantum Materials.
The findings could inform the design of practical superconducting devices.
Jennifer Chu | MIT News Office
Publication Date:July 8, 2022
(more at link)
When it comes to graphene, it appears that superconductivity runs in the family.
Graphene is a single-atom-thin material that can be exfoliated from the same graphite that is found in pencil lead. The ultrathin material is made entirely from carbon atoms that are arranged in a simple hexagonal pattern, similar to that of chicken wire. Since its isolation in 2004, graphene has been found to embody numerous remarkable properties in its single-layer form.
In 2018, MIT researchers found that if two graphene layers are stacked at a very specific “magic” angle, the twisted bilayer structure could exhibit robust superconductivity, a widely sought material state in which an electrical current can flow through with zero energy loss. Recently, the same group found a similar superconductive state exists in twisted trilayer graphene — a structure made from three graphene layers stacked at a precise, new magic angle.
Now the team reports that — you guessed it — four and five graphene layers can be twisted and stacked at new magic angles to elicit robust superconductivity at low temperatures. This latest discovery, published this week in Nature Materials, establishes the various twisted and stacked configurations of graphene as the first known “family” of multilayer magic-angle superconductors. The team also identified similarities and differences between graphene family members.
The findings could serve as a blueprint for designing practical, room-temperature superconductors. If the properties among family members could be replicated in other, naturally conductive materials, they could be harnessed, for instance, to deliver electricity without dissipation or build magnetically levitating trains that run without friction.
“The magic-angle graphene system is now a legitimate ‘family,’ beyond a couple of systems,” says lead author Jeong Min (Jane) Park, a graduate student in MIT’s Department of Physics. “Having this family is particularly meaningful because it provides a way to design robust superconductors.”
Park’s MIT co-authors include Yuan Cao, Li-Qiao Xia, Shuwen Sun, and Pablo Jarillo-Herrero, the Cecil and Ida Green Professor of Physics, along with Kenji Watanabe and Takashi Taniguchi of the National Institute for Materials Science in Tsukuba, Japan.
“No limit”
Jarillo-Herrero’s group was the first to discover magic-angle graphene, in the form of a bilayer structure of two graphene sheets placed one atop the other and slightly offset at a precise angle of 1.1 degrees. This twisted configuration, known as a moiré superlattice, transformed the material into a strong and persistent superconductor at ultralow temperatures.
The researchers also found that the material exhibited a type of electronic structure known as a “flat band,” in which the material’s electrons have the same energy, regardless of their momentum. In this flat band state, and at ultracold temperatures, the normally frenetic electrons collectively slow down enough to pair up in what are known as Cooper pairs — essential ingredients of superconductivity that can flow through the material without resistance.
While the researchers observed that twisted bilayer graphene exhibited both superconductivity and a flat band structure, it wasn’t clear whether the former arose from the latter.
“There was no proof a flat band structure led to superconductivity,” Park says. “Other groups since then have produced other twisted structures from other materials that have some flattish band, but they didn’t really have robust superconductivity. So we wondered: Could we produce another flat band superconducting device?”
As they considered this question, a group from Harvard University derived calculations that confirmed mathematically that three graphene layers, twisted at 1.6 degrees, would exhibit also flat bands, and suggested they may superconduct. They went on to show there should be no limit to the number of graphene layers that exhibit superconductivity, if stacked and twisted in just the right way, at angles they also predicted. Finally, they proved they could mathematically relate every multilayer structure to a common flat band structure — strong proof that a flat band may lead to robust superconductivity.
“They worked out there may be this entire hierarchy of graphene structures, to infinite layers, that might correspond to a similar mathematical expression for a flat band structure,” Park says.
Shortly after that work, Jarillo-Herrero’s group found that, indeed, superconductivity and a flat band emerged in twisted trilayer graphene — three graphene sheets, stacked like a cheese sandwich, the middle cheese layer shifted by 1.6 degrees with respect to the sandwiched outer layers. But the trilayer structure also showed subtle differences compared to its bilayer counterpart.
“That made us ask, where do these two structures fit in terms of the whole class of materials, and are they from the same family?” Park says.
An unconventional family
In the current study, the team looked to level up the number of graphene layers. They fabricated two new structures, made from four and five graphene layers, respectively. Each structure is stacked alternately, similar to the shifted cheese sandwich of twisted trilayer graphene.
The team kept the structures in a refrigerator below 1 kelvin (about -273 degrees Celsius), ran electrical current through each structure, and measured the output under various conditions, similar to tests for their bilayer and trilayer systems.
Overall, they found that both four- and five-layer twisted graphene also exhibit robust superconductivity and a flat band. The structures also shared other similarities with their three-layer counterpart, such as their response under a magnetic field of varying strength, angle, and orientation.
These experiments showed that twisted graphene structures could be considered a new family, or class of common superconducting materials. The experiments also suggested there may be a black sheep in the family: The original twisted bilayer structure, while sharing key properties, also showed subtle differences from its siblings. For instance, the group’s previous experiments showed the structure’s superconductivity broke down under lower magnetic fields and was more uneven as the field rotated, compared to its multilayer siblings.
The team carried out simulations of each structure type, seeking an explanation for the differences between family members. They concluded that the fact that twisted bilayer graphene’s superconductivity dies out under certain magnetic conditions is simply because all of its physical layers exist in a “nonmirrored” form within the structure. In other words, there are no two layers in the structure that are mirror opposites of each other, whereas graphene’s multilayer siblings exhibit some sort of mirror symmetry. These findings suggest that the mechanism driving electrons to flow in a robust superconductive state is the same across the twisted graphene family.
“That’s quite important,” Park notes. “Without knowing this, people might think bilayer graphene is more conventional compared to multilayer structures. But we show that this entire family may be unconventional, robust superconductors.”
This research was supported, in part, by the U.S. Department of Energy, the National Science Foundation, the Air Force Office of Scientific Research, the Gordon and Betty Moore Fundation, the Ramon Areces Foundation, and the CIFAR Program on Quantum Materials.
Chromium6- Posts : 729
Join date : 2019-11-29
Re: Partial List of Superconductors to Build Out
Unusual Superconductivity Observed in Twisted Trilayer Graphene
July 07, 2022
https://www.science.org/doi/10.1126/sciadv.abm9896
The ability to turn superconductivity off and on with a literal flip of a switch in so-called "magic-angle twisted graphene" has allowed engineers at Caltech to observe an unusual phenomenon that may shed new light on superconductivity in general.
The research, led by Stevan Nadj-Perge, assistant professor of applied physics and materials science, was published in the journal Nature on June 15.
Magic-angle twisted graphene, first discovered in 2018, is made from two or three sheets of graphene (a form of carbon consisting of a single layer of atoms in a honeycomb-like lattice pattern) layered atop one another, with each sheet twisted at precisely 1.05 degrees in relation to the one below it. The resulting bilayer or trilayer has unusual electronic properties: for example, it can be made into an insulator or a superconductor depending on how many electrons are added.
Superconductors are materials that exhibit a peculiar electronic state in which electrons can flow freely through the materials without resistance—meaning that electricity flows through them without any loss of energy to heat. Such hyper-efficient transmission of electricity has endless potential applications in the fields of computing, electronics, and elsewhere.
However, the catch with superconducting is that in most materials, it takes place at extremely low temperatures, usually only a few degrees above absolute zero (−273.15 degrees Celsius). At such temperatures, electrons forms pairs that behave in a fundamentally different way compared to individual electrons and condense into a quantum mechanical state that allows for electron pairs to flow without being scattered.
Superconductivity was first discovered more than a century ago, yet scientists still do not fully understand the precise mechanisms behind electron-pair formation for some materials. In conventional superconductors, such as metal aluminum, it is well understood that the attraction between electrons that leads to the formation of electron pairs is due to the interaction of the electrons with the material's crystal lattice. The behavior of these materials is described using the Bardeen–Cooper–Schrieffer (BCS) theory, named after John Bardeen, Leon Cooper, and John Robert Schrieffer, who shared the Nobel Prize in Physics in 1972 for the theory's development.
While studying magic-angle twisted trilayers of graphene, Nadj-Perge and his colleagues discovered that superconductivity in this material exhibits several very unusual properties that cannot be described using BSC theory, making it likely also an unconventional superconductor.
They measured the evolution of the so-called superconducting gap as the electrons are removed from the trilayer with the flip of a switch to turn an electric field on or off. The superconducting gap is a property that describes how difficult it is to add or remove individual electrons into a superconductor. Because electrons in a superconductor want to be paired, a certain amount of energy is required to break those pairs. However, the amount of energy can be different for pairs moving in different directions relative to the crystal lattice. As a result, the "gap" has a specific shape that is determined by the likelihood that pairs will be broken by a particular amount of energy.
"While superconductors have been around for a long time, a remarkably new feature in twisted graphene bilayers and trilayers is that superconductivity in these materials can be turned on simply through the application of a voltage on a nearby electrode," says Nadj-Perge, corresponding author of the Nature paper. "An electric field effectively adds or removes extra electrons. It works in a very similar way as the current is controlled in conventional transistors, and this allowed us to explore superconductivity in ways that one cannot do in other materials."
The team established that in twisted trilayers, two superconductivity regimes with differently shaped superconducting gap profiles are present. While one of the regimes can perhaps be explained with a theory that is to some extent similar to BCS, the presence of two regimes shows that within the superconducting phase an additional transition is likely to take place. This observation, alongside measurements taken at various temperatures and magnetic fields, points to the unconventional nature of superconductivity in the trilayers.
The new insights by Nadj-Perge's team give essential clues for the future theories of superconductivity in twisted graphene multilayers. Nadj-Perge notes that it appears that more layers make superconductivity more robust while remaining highly tunable, a property that opens up various possibilities to use twisted trilayers for superconducting devices that may someday be used in quantum science and perhaps quantum information processing.
"Besides its fundamental implications to our understanding of superconductivity, it is remarkable that adding an extra graphene layer made studying superconducting properties easier. Ultimately this is what enabled our findings," Nadj-Perge says.
The paper is titled "Evidence of unconventional superconductivity in twisted trilayer graphene." Co-authors include Jason Alicea, William K. Davis Professor of Theoretical Physics; Caltech graduate students Hyunjin Kim and Youngjoon Choi, leading authors on the paper; graduate students Robert Polski and Yiran Zhang; Cyprian Lewandowski, Moore Postdoctoral Scholar in Theoretical Physics; and Alex Thomson, Sherman Fairchild Postdoctoral Scholar in Theoretical Physics, who is now an assistant professor at UC Davis; and Kenji Watanabe and Takashi Taniguchi of the National Institute for Materials Science in Japan.
This research was funded by the National Science Foundation, the Office of Naval Research, the United States Department of Energy, the Kavli Nanoscience Institute, the Institute for Quantum Information and Matter at Caltech, the Walter Burke Institute for Theoretical Physics at Caltech, and the Kwanjeong Educational Foundation.
Image: The surface of a trilayer of graphene, as imaged by a scanning tunneling microscope. Due to the twist of a second layer, trilayer height is modulated with a period of about 9 nanometers.
https://www.caltech.edu/about/news/unusual-superconductivity-observed-in-twisted-trilayer-graphene
July 07, 2022
https://www.science.org/doi/10.1126/sciadv.abm9896
The ability to turn superconductivity off and on with a literal flip of a switch in so-called "magic-angle twisted graphene" has allowed engineers at Caltech to observe an unusual phenomenon that may shed new light on superconductivity in general.
The research, led by Stevan Nadj-Perge, assistant professor of applied physics and materials science, was published in the journal Nature on June 15.
Magic-angle twisted graphene, first discovered in 2018, is made from two or three sheets of graphene (a form of carbon consisting of a single layer of atoms in a honeycomb-like lattice pattern) layered atop one another, with each sheet twisted at precisely 1.05 degrees in relation to the one below it. The resulting bilayer or trilayer has unusual electronic properties: for example, it can be made into an insulator or a superconductor depending on how many electrons are added.
Superconductors are materials that exhibit a peculiar electronic state in which electrons can flow freely through the materials without resistance—meaning that electricity flows through them without any loss of energy to heat. Such hyper-efficient transmission of electricity has endless potential applications in the fields of computing, electronics, and elsewhere.
However, the catch with superconducting is that in most materials, it takes place at extremely low temperatures, usually only a few degrees above absolute zero (−273.15 degrees Celsius). At such temperatures, electrons forms pairs that behave in a fundamentally different way compared to individual electrons and condense into a quantum mechanical state that allows for electron pairs to flow without being scattered.
Superconductivity was first discovered more than a century ago, yet scientists still do not fully understand the precise mechanisms behind electron-pair formation for some materials. In conventional superconductors, such as metal aluminum, it is well understood that the attraction between electrons that leads to the formation of electron pairs is due to the interaction of the electrons with the material's crystal lattice. The behavior of these materials is described using the Bardeen–Cooper–Schrieffer (BCS) theory, named after John Bardeen, Leon Cooper, and John Robert Schrieffer, who shared the Nobel Prize in Physics in 1972 for the theory's development.
While studying magic-angle twisted trilayers of graphene, Nadj-Perge and his colleagues discovered that superconductivity in this material exhibits several very unusual properties that cannot be described using BSC theory, making it likely also an unconventional superconductor.
They measured the evolution of the so-called superconducting gap as the electrons are removed from the trilayer with the flip of a switch to turn an electric field on or off. The superconducting gap is a property that describes how difficult it is to add or remove individual electrons into a superconductor. Because electrons in a superconductor want to be paired, a certain amount of energy is required to break those pairs. However, the amount of energy can be different for pairs moving in different directions relative to the crystal lattice. As a result, the "gap" has a specific shape that is determined by the likelihood that pairs will be broken by a particular amount of energy.
"While superconductors have been around for a long time, a remarkably new feature in twisted graphene bilayers and trilayers is that superconductivity in these materials can be turned on simply through the application of a voltage on a nearby electrode," says Nadj-Perge, corresponding author of the Nature paper. "An electric field effectively adds or removes extra electrons. It works in a very similar way as the current is controlled in conventional transistors, and this allowed us to explore superconductivity in ways that one cannot do in other materials."
The team established that in twisted trilayers, two superconductivity regimes with differently shaped superconducting gap profiles are present. While one of the regimes can perhaps be explained with a theory that is to some extent similar to BCS, the presence of two regimes shows that within the superconducting phase an additional transition is likely to take place. This observation, alongside measurements taken at various temperatures and magnetic fields, points to the unconventional nature of superconductivity in the trilayers.
The new insights by Nadj-Perge's team give essential clues for the future theories of superconductivity in twisted graphene multilayers. Nadj-Perge notes that it appears that more layers make superconductivity more robust while remaining highly tunable, a property that opens up various possibilities to use twisted trilayers for superconducting devices that may someday be used in quantum science and perhaps quantum information processing.
"Besides its fundamental implications to our understanding of superconductivity, it is remarkable that adding an extra graphene layer made studying superconducting properties easier. Ultimately this is what enabled our findings," Nadj-Perge says.
The paper is titled "Evidence of unconventional superconductivity in twisted trilayer graphene." Co-authors include Jason Alicea, William K. Davis Professor of Theoretical Physics; Caltech graduate students Hyunjin Kim and Youngjoon Choi, leading authors on the paper; graduate students Robert Polski and Yiran Zhang; Cyprian Lewandowski, Moore Postdoctoral Scholar in Theoretical Physics; and Alex Thomson, Sherman Fairchild Postdoctoral Scholar in Theoretical Physics, who is now an assistant professor at UC Davis; and Kenji Watanabe and Takashi Taniguchi of the National Institute for Materials Science in Japan.
This research was funded by the National Science Foundation, the Office of Naval Research, the United States Department of Energy, the Kavli Nanoscience Institute, the Institute for Quantum Information and Matter at Caltech, the Walter Burke Institute for Theoretical Physics at Caltech, and the Kwanjeong Educational Foundation.
Image: The surface of a trilayer of graphene, as imaged by a scanning tunneling microscope. Due to the twist of a second layer, trilayer height is modulated with a period of about 9 nanometers.
https://www.caltech.edu/about/news/unusual-superconductivity-observed-in-twisted-trilayer-graphene
Last edited by Chromium6 on Fri Dec 02, 2022 6:20 am; edited 1 time in total
Chromium6- Posts : 729
Join date : 2019-11-29
Re: Partial List of Superconductors to Build Out
https://www.science.org/doi/10.1126/sciadv.abm9896
Supercurrent parity meter in a nanowire Cooper pair transistor
JI-YIN WANG HTTPS://ORCID.ORG/0000-0001-6783-7217, CONSTANTIN SCHRADE, VUKAN LEVAJAC, DAVID VAN DRIEL, KONGYI LI HTTPS://ORCID.ORG/0000-0003-1925-9808, SASA GAZIBEGOVIC HTTPS://ORCID.ORG/0000-0002-2740-9688, GHADA BADAWY HTTPS://ORCID.ORG/0000-0001-9933-3435, ROY L. M. OP HET VELD HTTPS://ORCID.ORG/0000-0001-9142-1422, JOON SUE LEE HTTPS://ORCID.ORG/0000-0002-1001-1462, [...], AND JIE SHEN HTTPS://ORCID.ORG/0000-0002-7205-5081 +6 authorsAuthors Info &
Abstract
We study a Cooper pair transistor realized by two Josephson weak links that enclose a superconducting island in an InSb-Al hybrid nanowire. When the nanowire is subject to a magnetic field, isolated subgap levels arise in the superconducting island and, because of the Coulomb blockade, mediate a supercurrent by coherent cotunneling of Cooper pairs. We show that the supercurrent resulting from such cotunneling events exhibits, for low to moderate magnetic fields, a phase offset that discriminates even and odd charge ground states on the superconducting island. Notably, this phase offset persists when a subgap state approaches zero energy and, based on theoretical considerations, permits parity measurements of subgap states by supercurrent interferometry. Such supercurrent parity measurements could, in a series of experiments, provide an alternative approach for manipulating and protecting quantum information stored in the isolated subgap levels of superconducting islands.
INTRODUCTION
When two superconducting (SC) leads couple via a Coulomb-blockaded quantum dot (QD), the isolated energy levels on the dot mediate a supercurrent by coherent cotunneling of Cooper pairs (1). For the case of a single-level QD, a control knob for the supercurrent direction is given by the charge parity of dot electrons (1). Such a parity-controlled supercurrent has been observed in a nanowire (NW) QD Josephson junction (JJ) (2, 3). It is described by the Josephson relation, I = (−1)n0Ic sin (φ), where Ic is the critical current, φ is the SC phase difference, and n0 is the number of dot electrons. In general, the Josephson relation can also acquire a phase offset, φ → φ + φ0 with φ0 ≠ 0, π, when time-reversal symmetry and mirror symmetry are broken (4). This breaking occurs, for example, if a spin-orbit coupled QD is subject to a magnetic field (4–7).
A different possibility of coupling two SC leads is via an SC island with finite charging energy: a “Cooper pair transistor” (CPT) (8–14). Unlike in the QD JJ, the SC island carries, within its parity lifetime, an even number of electrons in the ground state, as signified by a charging energy that is a 2e periodic function of the island gate charge (e, elementary charge) (10–12). In particular, since the odd charge states are energetically unfavorable for a conventional CPT, the Josephson relation is not expected to exhibit a parity-controlled phase offset.
Recently, a CPT has been realized with an indium arsenide–aluminum (Al) hybrid NW (12, 13). In this case, upon increasing a magnetic field parallel to the NW, a transition from a 2e periodic switching current to a switching current with even-odd pattern has been observed (12). The interpretation is that a low-energy subgap state arises in the SC island, and, depending on its occupancy, the charge ground state carries an even or an odd number of electrons. An open question is if the Josephson relation of an NW CPT exhibits in the presence of subgap states a parity-controlled phase offset.
Here, we address this question with an NW CPT integrated in a superconducting quantum interference device (SQUID). We investigate the previously described situation when the NW CPT is subject to a parallel magnetic field so that subgap levels arise in the SC island and mediate a supercurrent by coherent cotunneling of Cooper pairs. We show that supercurrent resulting from Cooper pair cotunneling exhibits a phase offset, which distinguishes even and odd charge ground states on the SC island. This phase offset persists when a subgap state approaches zero energy and, based on theoretical considerations, may enable parity readout of low-energy subgap states. Such supercurrent parity readout could provide a new approach for manipulating (15–20) and protecting (21, 22) quantum information stored in the isolated subgap levels of SC islands (23–27).
RESULTS
The device geometry of our experiment is shown in Fig. 1. For realizing the CPT, we use a shadow-grown (28) Al SC island on an indium antimonide (InSb) NW, which couples to two SC Al leads via gate-tunable tunneling barriers. A plunger gate is used for controlling the electron number on the SC island. As we intend to study the full Josephson relation of the NW CPT, we integrate our setup in a SQUID loop made of niobium titanium nitride (NbTiN) and a second InSb NW reference junction. The tunnel coupling of the reference junction is adjustable by a local gate electrode. Concrete fabrication steps are described in Methods.
Fig. 1. Sketch of the SQUID device.
(A) False-color micrograph of the measured NbTiN (green) SQUID device comprising an InSb-Al NW CPT in the right arm and an InSb nanowire reference junction in the left arm. Top gates (L, R, and REF) define tunable JJs, and a plunger gate (P) controls the electron number on the hybrid island. The InSb nanowires are ∼100 nm in diameter, Al shell is ∼10 nm in thickness, three junctions are ∼150 nm in length, and the InSb-Al hybrid island is ∼1 μm in length. (B) Cross sections along the lines shown in (A).
Initially, we pinch off the reference junction and characterize the NW CPT by measuring the differential conductance dI/dV versus the source-drain voltage V and the plunger gate voltage VP. Our results are shown in Fig. 2A for zero and finite parallel magnetic fields B∥.
Fig. 2. Parity control with magnetic field.
(A) Differential conductance, dI/dV, versus source-drain voltage V and plunger gate voltage VP. At zero parallel magnetic field, the differential conductance shows a Coulomb diamond pattern with a 2e periodicity. At B|| = 100 mT, the 2e periodicity of the Coulomb diamonds lifts because of the appearance of an odd-parity charge ground state on the SC island. Inset curves show the differential conductance at zero bias. Black dotted lines mark the boundary of a 2e charge Coulomb diamond at B|| = 0 and the boundary of an even-parity Coulomb diamond at B|| = 100 mT. (B) Top: Switching current, Isw, versus parallel magnetic field B|| and plunger gate voltage VP. Bottom: Magnetic field dependence of the normalized even and odd peak spacings, Se/(Se + So) and So/(Se + So), showing a transition from a 2e periodicity to an even-odd pattern.
At zero magnetic field, we observe a pattern of Coulomb diamonds with sharp edges due to the weak island-lead coupling. Besides the Coulomb diamonds, which signify the importance of charging effects on the SC island, the zero-bias differential conductance exhibits 2e periodic oscillations, which implies the transport of Cooper pairs (see the inset curve in Fig. 2A). Furthermore, above an onset voltage Vonset, a 1e periodic modulation of the differential conductance appears, which marks the onset of quasiparticle transport. The charging energy, EC, is estimated to be ∼20 μeV from the 2e charge diamond at B∥ = 0, and the induced gap, Δind, is extracted to be ∼50 μeV from onset of quasiparticle transport. The relation EC < Δind is consistent with the condition for 2e periodicity of the Coulomb diamonds at zero field (29–31).
At finite magnetic fields, the aforementioned onset voltage for quasiparticle transport persists. However, below the onset voltage, the Coulomb diamonds split, resulting in an even-odd pattern. We attribute the appearance of this even-odd pattern to low-energy subgap states that form on the SC island. More specifically, the magnetic field induces a Zeeman splitting of spinful, odd-parity states and, thereby, reduces the minimum single-particle excitation energy in the NW CPT. As a result, odd-parity states can detach from the quasiparticle continuum and, because of their enhanced effective g-factor in comparison to the Al shell, form isolated levels below the SC gap (12, 32).
Next, we investigate the subgap levels on the SC island in more detail. We lower the island-lead tunneling barriers and, with the reference junction still pinched off, measure the switching current Isw as a function of the parallel magnetic field B∥ and plunger gate voltage VP. Our results are depicted in Fig. 2B. At zero magnetic field, the switching current exhibits a 2e periodic peak spacing implying that the SC island always carries an even number of electrons in its charge ground state (see also fig. S1A). The situation changes upon applying a parallel magnetic field. The magnetic field induces a splitting of the 2e periodic peaks, and, as a result, the switching current exhibits a peak spacing with an even-odd pattern (see also fig. S1B). Similar to the differential conductance, we attribute the appearance of this even-odd pattern to charge ground states with even and odd fermion parity on the SC island. Moreover, as shown in Fig. 2B, the extracted peak spacings oscillate as a function of applied magnetic field, as well as the plunger gate voltage, indicating either the anticrossing or the crossing of the lowest-energy subgap state with a second subgap state at higher energy (30, 31).
We now open the reference junction and measure the NW CPT’s full Josephson relation in the presence of low-energy subgap states. For the results presented here, we focus on the magnetic field strength B∥ = 170 mT and adopt a highly asymmetric SQUID configuration so that the phase drop occurs primarily across the NW CPT. Under these conditions, we apply a bias current Ib and measure the voltage drop V across the SQUID as a function of the plunger gate voltage VP and the flux ϕ piercing through the SC loop. Figure 3 shows our measurement data, which we will now discuss in more detail.
Fig. 3. Superconducting phase difference between even and odd parities.
(A) Voltage drop V across the NW CPT versus applied bias current Ib and plunger gate voltage VP, showing an even-odd pattern consistent with the appearance of low-energy subgap states at a parallel magnetic field B|| = 170 mT. (B) Voltage drop V as a function of the applied bias current Ib and the flux ϕ that pierces through the SQUID loop for the plunger gate voltages VP marked in (A). The fitted switching current Isw (yellow) displays a phase offset φ0 that discriminates the even and odd charge parity sectors of the SC island. (C) Phase offset φ0 (relative to the even Coulomb valleys) versus plunger gate voltage VP. The dashed lines do not represent data but are merely used for improving data visibility. In the range of plunger gate voltages shown here, the phase offset is insensitive.
Our main finding is that the Josephson relation of the NW CPT exhibits a substantial relative phase offset φ0 between Coulomb valleys of opposite charge parity. To determine this phase offset for the Coulomb valleys marked in Fig. 3A, we fit the switching current Isw as a function of the flux ϕ. The fitted curves, shown in Fig. 3B, allow us to extract φ0 ∼ −1.24π and φ0 ∼ −1.31π for the first and second pairs of Coulomb valleys, respectively. For the remaining pairs, we find similar values for the phase offset, as summarized in Fig. 3C. Notably, the leftmost pair of data points in Fig. 3C shows that phase offset persists when the Coulomb peaks are close to a 1e spacing (see detailed analysis in fig. S2). Therefore, the phase offset facilitates charge parity readout even if a subgap state is close to zero energy.
Next, we discuss a possible mechanism for a parity-dependent phase offset. We introduce a model for the NW CPT, which comprises a mesoscopic SC island coupled to a pair of s-wave SC leads. In our model, we focus on the two lowest isolated subgap levels in the SC island, ±εa and ±εb, indicated by the peak spacing oscillation as a function of magnetic field and plunger gate in Fig. 2B. Here, we consider two types of cotunneling sequences:
1. In the first type of sequence, shown in Fig. 4A, the Cooper pair splits so that one electron tunnels via ±εa, while the other electron tunnels via ±εb. For such a two-level sequence, the corresponding supercurrent contribution acquires a prefactor given by the SC island charge parity, (−1)n0. This parity prefactor is analogous to the parity prefactor appearing in the Josephson relation of a QD JJ, where Cooper pairs tunnel via two dot levels with opposite spin polarization (1).
Fig. 4. Energy diagrams illustrating Cooper pair transport via subgap levels.
(A) Typical sequence of intermediate states in which a Cooper pair tunnels between the SC leads (left and right) via the two lowest isolated subgap levels a and b in the intermediate SC island (center). Such a sequence yields a contribution to the supercurrent proportional to the joint parity of the two subgap levels. In the illustration, numbers indicate the sequence of tunneling events, and solid/empty dots represent filled/empty subgap levels. The occupation numbers of the subgap levels (na and nb) in the sequence are (1,0)→1(0,0)→2(1,0)→3(1,1)→4(1,0). The energy of the initial odd parity (1, 0) configuration is (−1)na+1εa + (−1)nb+1εb = εa − εb, which corresponds to the ground state provided that εb>εa. (B) Typical sequence of intermediate states that involves Cooper pair transport via a single subgap level yielding no parity-dependent prefactor. The occupation numbers for this sequence are (1,0)→1(0,0)→2(1,0)→3(0,0)→4(1,0). In (A) and (B), subgap levels are displayed in an “excitation picture” representation (33).
2. In the second type of sequence, shown in Fig. 4B, both Cooper pair electrons tunnel via either ±εa or ±εb. For such a single-level sequence, each of the two electrons contributes a prefactor given by the parity of ±εa or ±εb. In particular, since the same parity prefactor appears twice in the sequence, it squares to one. Consequently, in the single-level supercurrent contribution, a parity prefactor is absent.
If we collect all sequences, we obtain the Josephson relation (see details in section 4 of the Supplementary Materials)
I=(−1)n0Iabsin (φ+φab)+∑ℓ=a,bIℓsin (φ+φℓ)
(1)
Here, Iab and Iℓ are amplitudes, which are 1e periodic in the gate charge if the lowest subgap level is at zero energy. Furthermore, the phase offsets φℓ arise if the subgap states couple inequivalently to the SC leads (see equation 17 in the Supplementary Materials for the detailed condition on the tunneling amplitudes) and if, due to time-reversal symmetry breaking, the tunnel couplings acquire complex phase factors.
We now highlight two differences between the NW CPT and a QD JJ: First, the island which mediates the Josephson current is in an SC state, not a normal state as for a QD JJ. Consequently, not only conventional tunneling events can occur but also anomalous tunneling events in which an electron is created/destroyed on both the SC island and the leads. Second, for a QD JJ, the wave functions on the dot are highly localized, which justifies a point-like tunneling contact. In comparison, for an NW CPT, the subgap level wave functions can be extended, which induces longer-range island-lead tunnel couplings. In particular, such longer-range couplings can break the mirror symmetry, due to the combined effect of spin-orbit coupling and magnetic field in the tunneling region, and lead to additional contributions to φab, φℓ.
Returning to Eq. 1, the total phase offset is φn0 ≡ arg [(−1)n0Iabeiφab + ∑ℓIℓeiφℓ], and the relative phase offset between the parity sectors is φ0 ≡ φn0+1 − φn0. In these expressions, the parity prefactor flips upon tuning the gate charge of the SC island between different charge parity sectors. As a result of these parity flips, the phase offset does not exhibit a 1e periodicity in the gate charge even if one of the subgap states is at zero energy. Instead, if Iab ≠ 0, φ0 is always 2e periodic and permits the measurement of the parity of the lowest subgap level. To practically enable such parity measurements, the two-level contribution should be sizable, Iab ≫ Iℓ. Also, to avoid thermal excitations, the temperature T should be small compared to the level separation ∣εa − εb∣. Therefore interestingly, if ∣εa − εb ∣ ≳ T, the parity prefactor measures the joint parity of ±εa and ±εb. Such joint parity measurements could be leveraged for entangling qubits stored in the subgap levels of SC islands (15–20).
So far, we have discussed a regime with substantial φ0 for parity readout with maximal resolution. However, such an ideal situation is not always realized. In Fig. 5A, we display the phase offset versus plunger gate voltage for multiple magnetic field values. For a selection of data points, we also show the fitted switching current Isw in Fig. 5B. Detailed analysis is shown in figs. S3 to S5. In comparison, there is another regime in which NW CPT exhibits phase independence on its parity (see details in figs. S6 and S7). In Fig. 5, our findings are twofold: First, we observe that the phase offset for subsequent Coulomb valley pairs is tunable by the magnetic field and the plunger gate voltage. Such a tunability arises because both control parameters change the support of the subgap level wave function and, thereby, alter the lead-island Josephson couplings. Second, we find that the phase offset decreases upon increasing the magnetic field. This decrease suggests that the level separation between the lowest-energy and higher-energy subgap states increases so that the supercurrent contribution with the parity-dependent prefactor becomes energetically unfavorable. As a result, in this regime, the NW CPT exhibits a phase dependence that is only weakly dependent on its parity.
Fig. 5. Tunable phase offset.
(A) Phase offset φ0 versus plunger gate voltage VP for various parallel magnetic fields B||. The dashed lines do not represent data but are merely used for improving data visibility. The phase offset is sensitive to both plunger gate voltage and magnetic field variations. (B) Voltage drop V as a function of the applied bias current Ib and the SQUID flux ϕ for a parallel magnetic field B|| = 160 mT. The switching current Isw (yellow) displays a phase offset φ0 between even (e) and odd (o) Coulomb valleys of the SC island that is tunable by the plunger gate voltage VP.
DISCUSSION
In summary, we have studied the Josephson relation of an InSb-Al NW CPT. We have demonstrated that upon applying a magnetic field, subgap levels arise in the SC island and mediate a supercurrent with a parity-dependent phase offset. We have shown that the phase offset persists when the subgap state approaches zero energy and enables parity readout of the lowest energy subgap state. Such a supercurrent parity readout could be useful for the manipulation (15–20) and protection (21, 22) of qubits stored in the isolated subgap levels of SC islands (23–27).
METHODS
Device fabrication
The InSb NWs used in the experiment were grown on an indium phosphide substrate by metalorganic vapor-phase epitaxy. In the molecular beam epitaxy chamber, Al flux was deposited along a specific direction to form Al shadows on InSb NWs by neighboring NWs (28). InSb-Al NWs with shadows were transferred onto a doped Si/SiOx substrate using a nanomanipulator installed inside a scanning electron microscope. NbTiN superconductor was sputter deposited right after Ar etching dedicated to removing the oxidized layer. Subsequently, 30-nm SiNx was sputter deposited to work as a dielectric layer, and 10/120-nm Ti/Au was used as a top gate.
Transport measurement
The sample was measured at a base temperature of ∼20 mK in an Oxford dry dilution refrigerator equipped with a vector magnet. Differential conductance was measured by applying small AC lock-in excitation superimposed on a DC voltage and then measuring AC and DC current through the device. Typically, low frequency of ∼27 Hz and AC excitation amplitude of ∼10 μV were used for lock-in measurement. In current bias measurement, current was applied through the device while monitoring voltage drop on device. The direction of the magnetic field was aligned with respect to the InSb-Al island arm by detecting the supercurrent of CPT while rotating the magnetic field direction.
Acknowledgments
We are grateful to R. Lutchyn, B. van Heck, M. de Moor, C.-X. Liu, and M. P. Vidal for the fruitful discussions.
Funding: J.S. acknowledges support from the National Science Foundation of China under grant nos. 12174430 and 92065203, Chinese Academy of Sciences under grant no. XDB33000000, the Beijing Nova Program under grant no. Z211100002121144, and the Synergic Extreme Condition User Facility. The work of G.B. has been supported by NWO (715.016.001). This work has been supported by the Dutch Organization for Scientific Research (NWO), the Foundation for Fundamental Research on Matter (FOM), and Microsoft Corporation Station Q.
Author contributions: J.-Y.W. and J.S. conceived the experiment. J.-Y.W. and J.S. fabricated the device. J.-Y.W., J.S., and D.v.D. performed the measurements. C.S. and L.F. did the theory simulations. K.L. helped transfer the nanowires. S.G., G.B., R.L.M.O.h.V., J.S.L., M.P., C.P.D., C.J.P., and E.P.A.M.B. carried out the material growth. J.-Y.W., J.S., C.S., V.L., and L.P.K. discussed and analyzed the data. C.S., J.S., and J.-Y.W. wrote manuscript with input from all authors.
Competing interests: The authors declare that they have no competing interests.
Data and materials availability: All data needed to evaluate the conclusions in the paper are present in the paper and/or the Supplementary Materials. The authors declare that all of the raw data together with analysis files are available at https://doi.org/10.5281/zenodo.5075186.
Supplementary Materials
This PDF file includes:
Supplementary Text
Figs. S1 to S7
References
Supercurrent parity meter in a nanowire Cooper pair transistor
JI-YIN WANG HTTPS://ORCID.ORG/0000-0001-6783-7217, CONSTANTIN SCHRADE, VUKAN LEVAJAC, DAVID VAN DRIEL, KONGYI LI HTTPS://ORCID.ORG/0000-0003-1925-9808, SASA GAZIBEGOVIC HTTPS://ORCID.ORG/0000-0002-2740-9688, GHADA BADAWY HTTPS://ORCID.ORG/0000-0001-9933-3435, ROY L. M. OP HET VELD HTTPS://ORCID.ORG/0000-0001-9142-1422, JOON SUE LEE HTTPS://ORCID.ORG/0000-0002-1001-1462, [...], AND JIE SHEN HTTPS://ORCID.ORG/0000-0002-7205-5081 +6 authorsAuthors Info &
Abstract
We study a Cooper pair transistor realized by two Josephson weak links that enclose a superconducting island in an InSb-Al hybrid nanowire. When the nanowire is subject to a magnetic field, isolated subgap levels arise in the superconducting island and, because of the Coulomb blockade, mediate a supercurrent by coherent cotunneling of Cooper pairs. We show that the supercurrent resulting from such cotunneling events exhibits, for low to moderate magnetic fields, a phase offset that discriminates even and odd charge ground states on the superconducting island. Notably, this phase offset persists when a subgap state approaches zero energy and, based on theoretical considerations, permits parity measurements of subgap states by supercurrent interferometry. Such supercurrent parity measurements could, in a series of experiments, provide an alternative approach for manipulating and protecting quantum information stored in the isolated subgap levels of superconducting islands.
INTRODUCTION
When two superconducting (SC) leads couple via a Coulomb-blockaded quantum dot (QD), the isolated energy levels on the dot mediate a supercurrent by coherent cotunneling of Cooper pairs (1). For the case of a single-level QD, a control knob for the supercurrent direction is given by the charge parity of dot electrons (1). Such a parity-controlled supercurrent has been observed in a nanowire (NW) QD Josephson junction (JJ) (2, 3). It is described by the Josephson relation, I = (−1)n0Ic sin (φ), where Ic is the critical current, φ is the SC phase difference, and n0 is the number of dot electrons. In general, the Josephson relation can also acquire a phase offset, φ → φ + φ0 with φ0 ≠ 0, π, when time-reversal symmetry and mirror symmetry are broken (4). This breaking occurs, for example, if a spin-orbit coupled QD is subject to a magnetic field (4–7).
A different possibility of coupling two SC leads is via an SC island with finite charging energy: a “Cooper pair transistor” (CPT) (8–14). Unlike in the QD JJ, the SC island carries, within its parity lifetime, an even number of electrons in the ground state, as signified by a charging energy that is a 2e periodic function of the island gate charge (e, elementary charge) (10–12). In particular, since the odd charge states are energetically unfavorable for a conventional CPT, the Josephson relation is not expected to exhibit a parity-controlled phase offset.
Recently, a CPT has been realized with an indium arsenide–aluminum (Al) hybrid NW (12, 13). In this case, upon increasing a magnetic field parallel to the NW, a transition from a 2e periodic switching current to a switching current with even-odd pattern has been observed (12). The interpretation is that a low-energy subgap state arises in the SC island, and, depending on its occupancy, the charge ground state carries an even or an odd number of electrons. An open question is if the Josephson relation of an NW CPT exhibits in the presence of subgap states a parity-controlled phase offset.
Here, we address this question with an NW CPT integrated in a superconducting quantum interference device (SQUID). We investigate the previously described situation when the NW CPT is subject to a parallel magnetic field so that subgap levels arise in the SC island and mediate a supercurrent by coherent cotunneling of Cooper pairs. We show that supercurrent resulting from Cooper pair cotunneling exhibits a phase offset, which distinguishes even and odd charge ground states on the SC island. This phase offset persists when a subgap state approaches zero energy and, based on theoretical considerations, may enable parity readout of low-energy subgap states. Such supercurrent parity readout could provide a new approach for manipulating (15–20) and protecting (21, 22) quantum information stored in the isolated subgap levels of SC islands (23–27).
RESULTS
The device geometry of our experiment is shown in Fig. 1. For realizing the CPT, we use a shadow-grown (28) Al SC island on an indium antimonide (InSb) NW, which couples to two SC Al leads via gate-tunable tunneling barriers. A plunger gate is used for controlling the electron number on the SC island. As we intend to study the full Josephson relation of the NW CPT, we integrate our setup in a SQUID loop made of niobium titanium nitride (NbTiN) and a second InSb NW reference junction. The tunnel coupling of the reference junction is adjustable by a local gate electrode. Concrete fabrication steps are described in Methods.
Fig. 1. Sketch of the SQUID device.
(A) False-color micrograph of the measured NbTiN (green) SQUID device comprising an InSb-Al NW CPT in the right arm and an InSb nanowire reference junction in the left arm. Top gates (L, R, and REF) define tunable JJs, and a plunger gate (P) controls the electron number on the hybrid island. The InSb nanowires are ∼100 nm in diameter, Al shell is ∼10 nm in thickness, three junctions are ∼150 nm in length, and the InSb-Al hybrid island is ∼1 μm in length. (B) Cross sections along the lines shown in (A).
Initially, we pinch off the reference junction and characterize the NW CPT by measuring the differential conductance dI/dV versus the source-drain voltage V and the plunger gate voltage VP. Our results are shown in Fig. 2A for zero and finite parallel magnetic fields B∥.
Fig. 2. Parity control with magnetic field.
(A) Differential conductance, dI/dV, versus source-drain voltage V and plunger gate voltage VP. At zero parallel magnetic field, the differential conductance shows a Coulomb diamond pattern with a 2e periodicity. At B|| = 100 mT, the 2e periodicity of the Coulomb diamonds lifts because of the appearance of an odd-parity charge ground state on the SC island. Inset curves show the differential conductance at zero bias. Black dotted lines mark the boundary of a 2e charge Coulomb diamond at B|| = 0 and the boundary of an even-parity Coulomb diamond at B|| = 100 mT. (B) Top: Switching current, Isw, versus parallel magnetic field B|| and plunger gate voltage VP. Bottom: Magnetic field dependence of the normalized even and odd peak spacings, Se/(Se + So) and So/(Se + So), showing a transition from a 2e periodicity to an even-odd pattern.
At zero magnetic field, we observe a pattern of Coulomb diamonds with sharp edges due to the weak island-lead coupling. Besides the Coulomb diamonds, which signify the importance of charging effects on the SC island, the zero-bias differential conductance exhibits 2e periodic oscillations, which implies the transport of Cooper pairs (see the inset curve in Fig. 2A). Furthermore, above an onset voltage Vonset, a 1e periodic modulation of the differential conductance appears, which marks the onset of quasiparticle transport. The charging energy, EC, is estimated to be ∼20 μeV from the 2e charge diamond at B∥ = 0, and the induced gap, Δind, is extracted to be ∼50 μeV from onset of quasiparticle transport. The relation EC < Δind is consistent with the condition for 2e periodicity of the Coulomb diamonds at zero field (29–31).
At finite magnetic fields, the aforementioned onset voltage for quasiparticle transport persists. However, below the onset voltage, the Coulomb diamonds split, resulting in an even-odd pattern. We attribute the appearance of this even-odd pattern to low-energy subgap states that form on the SC island. More specifically, the magnetic field induces a Zeeman splitting of spinful, odd-parity states and, thereby, reduces the minimum single-particle excitation energy in the NW CPT. As a result, odd-parity states can detach from the quasiparticle continuum and, because of their enhanced effective g-factor in comparison to the Al shell, form isolated levels below the SC gap (12, 32).
Next, we investigate the subgap levels on the SC island in more detail. We lower the island-lead tunneling barriers and, with the reference junction still pinched off, measure the switching current Isw as a function of the parallel magnetic field B∥ and plunger gate voltage VP. Our results are depicted in Fig. 2B. At zero magnetic field, the switching current exhibits a 2e periodic peak spacing implying that the SC island always carries an even number of electrons in its charge ground state (see also fig. S1A). The situation changes upon applying a parallel magnetic field. The magnetic field induces a splitting of the 2e periodic peaks, and, as a result, the switching current exhibits a peak spacing with an even-odd pattern (see also fig. S1B). Similar to the differential conductance, we attribute the appearance of this even-odd pattern to charge ground states with even and odd fermion parity on the SC island. Moreover, as shown in Fig. 2B, the extracted peak spacings oscillate as a function of applied magnetic field, as well as the plunger gate voltage, indicating either the anticrossing or the crossing of the lowest-energy subgap state with a second subgap state at higher energy (30, 31).
We now open the reference junction and measure the NW CPT’s full Josephson relation in the presence of low-energy subgap states. For the results presented here, we focus on the magnetic field strength B∥ = 170 mT and adopt a highly asymmetric SQUID configuration so that the phase drop occurs primarily across the NW CPT. Under these conditions, we apply a bias current Ib and measure the voltage drop V across the SQUID as a function of the plunger gate voltage VP and the flux ϕ piercing through the SC loop. Figure 3 shows our measurement data, which we will now discuss in more detail.
Fig. 3. Superconducting phase difference between even and odd parities.
(A) Voltage drop V across the NW CPT versus applied bias current Ib and plunger gate voltage VP, showing an even-odd pattern consistent with the appearance of low-energy subgap states at a parallel magnetic field B|| = 170 mT. (B) Voltage drop V as a function of the applied bias current Ib and the flux ϕ that pierces through the SQUID loop for the plunger gate voltages VP marked in (A). The fitted switching current Isw (yellow) displays a phase offset φ0 that discriminates the even and odd charge parity sectors of the SC island. (C) Phase offset φ0 (relative to the even Coulomb valleys) versus plunger gate voltage VP. The dashed lines do not represent data but are merely used for improving data visibility. In the range of plunger gate voltages shown here, the phase offset is insensitive.
Our main finding is that the Josephson relation of the NW CPT exhibits a substantial relative phase offset φ0 between Coulomb valleys of opposite charge parity. To determine this phase offset for the Coulomb valleys marked in Fig. 3A, we fit the switching current Isw as a function of the flux ϕ. The fitted curves, shown in Fig. 3B, allow us to extract φ0 ∼ −1.24π and φ0 ∼ −1.31π for the first and second pairs of Coulomb valleys, respectively. For the remaining pairs, we find similar values for the phase offset, as summarized in Fig. 3C. Notably, the leftmost pair of data points in Fig. 3C shows that phase offset persists when the Coulomb peaks are close to a 1e spacing (see detailed analysis in fig. S2). Therefore, the phase offset facilitates charge parity readout even if a subgap state is close to zero energy.
Next, we discuss a possible mechanism for a parity-dependent phase offset. We introduce a model for the NW CPT, which comprises a mesoscopic SC island coupled to a pair of s-wave SC leads. In our model, we focus on the two lowest isolated subgap levels in the SC island, ±εa and ±εb, indicated by the peak spacing oscillation as a function of magnetic field and plunger gate in Fig. 2B. Here, we consider two types of cotunneling sequences:
1. In the first type of sequence, shown in Fig. 4A, the Cooper pair splits so that one electron tunnels via ±εa, while the other electron tunnels via ±εb. For such a two-level sequence, the corresponding supercurrent contribution acquires a prefactor given by the SC island charge parity, (−1)n0. This parity prefactor is analogous to the parity prefactor appearing in the Josephson relation of a QD JJ, where Cooper pairs tunnel via two dot levels with opposite spin polarization (1).
Fig. 4. Energy diagrams illustrating Cooper pair transport via subgap levels.
(A) Typical sequence of intermediate states in which a Cooper pair tunnels between the SC leads (left and right) via the two lowest isolated subgap levels a and b in the intermediate SC island (center). Such a sequence yields a contribution to the supercurrent proportional to the joint parity of the two subgap levels. In the illustration, numbers indicate the sequence of tunneling events, and solid/empty dots represent filled/empty subgap levels. The occupation numbers of the subgap levels (na and nb) in the sequence are (1,0)→1(0,0)→2(1,0)→3(1,1)→4(1,0). The energy of the initial odd parity (1, 0) configuration is (−1)na+1εa + (−1)nb+1εb = εa − εb, which corresponds to the ground state provided that εb>εa. (B) Typical sequence of intermediate states that involves Cooper pair transport via a single subgap level yielding no parity-dependent prefactor. The occupation numbers for this sequence are (1,0)→1(0,0)→2(1,0)→3(0,0)→4(1,0). In (A) and (B), subgap levels are displayed in an “excitation picture” representation (33).
2. In the second type of sequence, shown in Fig. 4B, both Cooper pair electrons tunnel via either ±εa or ±εb. For such a single-level sequence, each of the two electrons contributes a prefactor given by the parity of ±εa or ±εb. In particular, since the same parity prefactor appears twice in the sequence, it squares to one. Consequently, in the single-level supercurrent contribution, a parity prefactor is absent.
If we collect all sequences, we obtain the Josephson relation (see details in section 4 of the Supplementary Materials)
I=(−1)n0Iabsin (φ+φab)+∑ℓ=a,bIℓsin (φ+φℓ)
(1)
Here, Iab and Iℓ are amplitudes, which are 1e periodic in the gate charge if the lowest subgap level is at zero energy. Furthermore, the phase offsets φℓ arise if the subgap states couple inequivalently to the SC leads (see equation 17 in the Supplementary Materials for the detailed condition on the tunneling amplitudes) and if, due to time-reversal symmetry breaking, the tunnel couplings acquire complex phase factors.
We now highlight two differences between the NW CPT and a QD JJ: First, the island which mediates the Josephson current is in an SC state, not a normal state as for a QD JJ. Consequently, not only conventional tunneling events can occur but also anomalous tunneling events in which an electron is created/destroyed on both the SC island and the leads. Second, for a QD JJ, the wave functions on the dot are highly localized, which justifies a point-like tunneling contact. In comparison, for an NW CPT, the subgap level wave functions can be extended, which induces longer-range island-lead tunnel couplings. In particular, such longer-range couplings can break the mirror symmetry, due to the combined effect of spin-orbit coupling and magnetic field in the tunneling region, and lead to additional contributions to φab, φℓ.
Returning to Eq. 1, the total phase offset is φn0 ≡ arg [(−1)n0Iabeiφab + ∑ℓIℓeiφℓ], and the relative phase offset between the parity sectors is φ0 ≡ φn0+1 − φn0. In these expressions, the parity prefactor flips upon tuning the gate charge of the SC island between different charge parity sectors. As a result of these parity flips, the phase offset does not exhibit a 1e periodicity in the gate charge even if one of the subgap states is at zero energy. Instead, if Iab ≠ 0, φ0 is always 2e periodic and permits the measurement of the parity of the lowest subgap level. To practically enable such parity measurements, the two-level contribution should be sizable, Iab ≫ Iℓ. Also, to avoid thermal excitations, the temperature T should be small compared to the level separation ∣εa − εb∣. Therefore interestingly, if ∣εa − εb ∣ ≳ T, the parity prefactor measures the joint parity of ±εa and ±εb. Such joint parity measurements could be leveraged for entangling qubits stored in the subgap levels of SC islands (15–20).
So far, we have discussed a regime with substantial φ0 for parity readout with maximal resolution. However, such an ideal situation is not always realized. In Fig. 5A, we display the phase offset versus plunger gate voltage for multiple magnetic field values. For a selection of data points, we also show the fitted switching current Isw in Fig. 5B. Detailed analysis is shown in figs. S3 to S5. In comparison, there is another regime in which NW CPT exhibits phase independence on its parity (see details in figs. S6 and S7). In Fig. 5, our findings are twofold: First, we observe that the phase offset for subsequent Coulomb valley pairs is tunable by the magnetic field and the plunger gate voltage. Such a tunability arises because both control parameters change the support of the subgap level wave function and, thereby, alter the lead-island Josephson couplings. Second, we find that the phase offset decreases upon increasing the magnetic field. This decrease suggests that the level separation between the lowest-energy and higher-energy subgap states increases so that the supercurrent contribution with the parity-dependent prefactor becomes energetically unfavorable. As a result, in this regime, the NW CPT exhibits a phase dependence that is only weakly dependent on its parity.
Fig. 5. Tunable phase offset.
(A) Phase offset φ0 versus plunger gate voltage VP for various parallel magnetic fields B||. The dashed lines do not represent data but are merely used for improving data visibility. The phase offset is sensitive to both plunger gate voltage and magnetic field variations. (B) Voltage drop V as a function of the applied bias current Ib and the SQUID flux ϕ for a parallel magnetic field B|| = 160 mT. The switching current Isw (yellow) displays a phase offset φ0 between even (e) and odd (o) Coulomb valleys of the SC island that is tunable by the plunger gate voltage VP.
DISCUSSION
In summary, we have studied the Josephson relation of an InSb-Al NW CPT. We have demonstrated that upon applying a magnetic field, subgap levels arise in the SC island and mediate a supercurrent with a parity-dependent phase offset. We have shown that the phase offset persists when the subgap state approaches zero energy and enables parity readout of the lowest energy subgap state. Such a supercurrent parity readout could be useful for the manipulation (15–20) and protection (21, 22) of qubits stored in the isolated subgap levels of SC islands (23–27).
METHODS
Device fabrication
The InSb NWs used in the experiment were grown on an indium phosphide substrate by metalorganic vapor-phase epitaxy. In the molecular beam epitaxy chamber, Al flux was deposited along a specific direction to form Al shadows on InSb NWs by neighboring NWs (28). InSb-Al NWs with shadows were transferred onto a doped Si/SiOx substrate using a nanomanipulator installed inside a scanning electron microscope. NbTiN superconductor was sputter deposited right after Ar etching dedicated to removing the oxidized layer. Subsequently, 30-nm SiNx was sputter deposited to work as a dielectric layer, and 10/120-nm Ti/Au was used as a top gate.
Transport measurement
The sample was measured at a base temperature of ∼20 mK in an Oxford dry dilution refrigerator equipped with a vector magnet. Differential conductance was measured by applying small AC lock-in excitation superimposed on a DC voltage and then measuring AC and DC current through the device. Typically, low frequency of ∼27 Hz and AC excitation amplitude of ∼10 μV were used for lock-in measurement. In current bias measurement, current was applied through the device while monitoring voltage drop on device. The direction of the magnetic field was aligned with respect to the InSb-Al island arm by detecting the supercurrent of CPT while rotating the magnetic field direction.
Acknowledgments
We are grateful to R. Lutchyn, B. van Heck, M. de Moor, C.-X. Liu, and M. P. Vidal for the fruitful discussions.
Funding: J.S. acknowledges support from the National Science Foundation of China under grant nos. 12174430 and 92065203, Chinese Academy of Sciences under grant no. XDB33000000, the Beijing Nova Program under grant no. Z211100002121144, and the Synergic Extreme Condition User Facility. The work of G.B. has been supported by NWO (715.016.001). This work has been supported by the Dutch Organization for Scientific Research (NWO), the Foundation for Fundamental Research on Matter (FOM), and Microsoft Corporation Station Q.
Author contributions: J.-Y.W. and J.S. conceived the experiment. J.-Y.W. and J.S. fabricated the device. J.-Y.W., J.S., and D.v.D. performed the measurements. C.S. and L.F. did the theory simulations. K.L. helped transfer the nanowires. S.G., G.B., R.L.M.O.h.V., J.S.L., M.P., C.P.D., C.J.P., and E.P.A.M.B. carried out the material growth. J.-Y.W., J.S., C.S., V.L., and L.P.K. discussed and analyzed the data. C.S., J.S., and J.-Y.W. wrote manuscript with input from all authors.
Competing interests: The authors declare that they have no competing interests.
Data and materials availability: All data needed to evaluate the conclusions in the paper are present in the paper and/or the Supplementary Materials. The authors declare that all of the raw data together with analysis files are available at https://doi.org/10.5281/zenodo.5075186.
Supplementary Materials
This PDF file includes:
Supplementary Text
Figs. S1 to S7
References
Chromium6- Posts : 729
Join date : 2019-11-29
Re: Partial List of Superconductors to Build Out
November 7, 2022
Shedding light on the superconductivity of newly-discovered kagome metals
by Duyen Nguyen, University of Pennsylvania
Liang Wu is an assistant professor in the Department of Physics and Astronomy at the School of Arts & Sciences. Credit: University of Pennsylvania
Already used in computers and MRI machines, superconductors—materials that can transmit electricity without resistance—hold promise for the development of even more advanced technologies, like hover trains and quantum computing. Yet, how superconductivity works in many materials remains a mystery that limits its applications.
A new study published in Nature Physics sheds light on the superconductivity of AV3Sb5, a recently discovered family of kagome metals. The research was led by Liang Wu of the School of Arts & Sciences and conducted by Yishuai Xu, a postdoc in Wu's lab, and graduate students Zhuoliang Ni and Qinwen Deng, in collaboration with researchers from the Weizmann Institute of Science and University of California, Santa Barbara.
Since their discovery, superconductors with the chemical formula AV3Sb5—where A refers to cesium, rubidium, or potassium—have generated immense interest for their exotic properties. The compounds feature a kagome lattice, an unusual atomic arrangement that resembles and takes its name from a Japanese basket-weave pattern of interlaced, corner-sharing triangles. Kagome lattice materials have fascinated researchers for decades because they provide a window into quantum phenomena such as geometrical frustration, topology, and strong correlations.
While previous research on AV3Sb5 has discovered the coexistence of two different cooperative electronic states—the charge-density wave order and superconductivity—the nature of the symmetry breaking that accompanies these states has been unclear. In physics, symmetry refers to a physical or mathematical feature of a system that remains unchanged under certain transformations. When a material transitions from a normal, high-temperature state to an exotic, low-temperature state like superconductivity, it undergoes symmetry breaking. Wu, whose lab develops and uses time-resolved and nonlinear optical techniques to study quantum materials, set out to clarify the nature of symmetry-breaking when AV3Sb5 enters the charge-density wave phase.
AV3Sb5 exhibits what researchers call a "cascade" of symmetry-broken phases. In other words, as the system cools down, it begins to enter a symmetry breaking state, with lower and lower temperatures leading to additional broken symmetries. "In order to use superconductors for applications, we need to understand them," Wu says. "Because superconductivity develops at even lower temperatures, we need to understand the charge-density wave phase first."
In its normal state, AV3Sb5 consists of a hexagonal crystal structure, composed of kagome lattices of vanadium (V) atoms coordinated by antimony (Sb) stacked on top of one another, with sheets of cesium, rubidium, or potassium in between each V-Sb layer. The structure is six-fold rotationally symmetric; when rotated by 60 degrees, it stays the same.
To find out whether AV3Sb5 retains its six-fold symmetry in the charge-density wave phase, the researchers performed scanning birefringence measurements on all three members of the AV3Sb5 family. Birefringence, or double refraction, refers to an optical property exhibited by materials with crystallographically distinct axes, a principal axis and a non-equivalent axis. When light enters the material along the non-equivalent axis, it splits in two, with each ray polarized and traveling at different speeds.
"In a kagome plane, the linear optical response should be the same along any direction, but they're not in AV3Sb5 because between the two kagome layers there's a relative shift," Wu says, explaining that the birefringence measurements revealed the difference between two orthogonal directions in the plane and a phase shift between the two layers that reduces the six-fold rotational symmetry of the materials to two-fold when they enter the charge-density wave state. "This was not clear to the physics community before."
Distinct axes are not the only explanation for the rotation of the light polarization plane. When linearly polarized light encounters a magnetic surface, it also changes, a phenomenon known as the magneto-optical Kerr effect. After separating out the property of birefringence by sending light along the principal axis in samples of AV3Sb5, the researchers used a second optical technique to measure the onset of the Kerr effect. For all three metals, the experiments reveal that the Kerr effect begins in the charge-density wave state. This finding indicates that the formation of charge-density waves breaks another symmetry, time-reversal symmetry. The simplest way to break time-reversal symmetry—which holds that the laws of physics remain the same whether time runs forward or backwards—is to use a permanent magnet, like those we put on a refrigerator, Wu says.
However, the Kerr effect is only observable at low temperatures with high resolution, indicating that the kagome metals are not substantially magnetic. "With these quantum materials," Wu says, he and his collaborators theorize that time-reversal symmetry is "not broken by a permanent magnet but by a circulating loop current." To confirm the nature of time-reversal symmetry breaking in the charge-density wave state, the researchers performed a third experiment in which they measured the circular dichroism, or the unequal reflectivity of left-handed and right-handed circularly polarized light, of the charge density wave phase. "We still need further work, but this finding really supports the possibility of circulating loop currents," the existence of which would suggest the unconventional nature of superconductivity in the metals, says Wu.
In 2018 Congress passed the National Quantum Initiative Act, with the goal of advancing research on quantum materials and the development of quantum technology. Quantum materials include those with topological properties and those with correlation, like the kagome metals AV3Sb5. While Wu's previous research centered on the former category and antiferromagnets, he says that the scanning optics technique that he'd developed for these studies presented a "ready and versatile tool" for studying symmetry breaking in new kagome metals.
More at link: https://phys.org/news/2022-11-superconductivity-newly-discovered-kagome-metals.html
Paper:
https://arxiv.org/abs/2109.10809v1
Kagome superconductors AV3Sb5 (A=K, Rb, Cs)
Kun Jiang, Tao Wu, Jia-Xin Yin, Zhenyu Wang, M. Zahid Hasan, Stephen D. Wilson, Xianhui Chen, Jiangping Hu
The quasi two-dimensional (quasi-2D) kagome materials AV3Sb5 (A=K, Rb, Cs) were found to be a prime example of kagome superconductors, a new quantum platform to investigate the interplay between electron correlation effects, topology and geometric frustration. In this review, we report recent progress on the experimental and theoretical studies of AV3Sb5 and provide a broad picture of this fast-developing field in order to stimulate an expanded search for unconventional kagome superconductors. We review the electronic properties of AV3Sb5, the experimental measurements of the charge density wave state, evidence of time-reversal symmetry breaking, and other potential hidden symmetry breaking in these materials. A variety of theoretical proposals and models that address the nature of the time-reversal symmetry breaking are discussed. Finally, we review the superconducting properties of AV3Sb5, especially the potential pairing symmetries and the interplay between superconductivity and the charge density wave state.
Comments: A review on kagome superconductors AV3Sb5, including 14 pages and 9 figures
Subjects: Superconductivity (cond-mat.supr-con); Materials Science (cond-mat.mtrl-sci); Strongly Correlated Electrons (cond-mat.str-el)
Cite as: arXiv:2109.10809 [cond-mat.supr-con]
(or arXiv:2109.10809v1 [cond-mat.supr-con] for this version)
https://doi.org/10.48550/arXiv.2109.10809
Related DOI:
https://doi.org/10.1093/nsr/nwac199
Submission history
From: Kun Jiang [view email]
[v1] Wed, 22 Sep 2021 16:01:25 UTC (8,644 KB)

Shedding light on the superconductivity of newly-discovered kagome metals
by Duyen Nguyen, University of Pennsylvania
Liang Wu is an assistant professor in the Department of Physics and Astronomy at the School of Arts & Sciences. Credit: University of Pennsylvania
Already used in computers and MRI machines, superconductors—materials that can transmit electricity without resistance—hold promise for the development of even more advanced technologies, like hover trains and quantum computing. Yet, how superconductivity works in many materials remains a mystery that limits its applications.
A new study published in Nature Physics sheds light on the superconductivity of AV3Sb5, a recently discovered family of kagome metals. The research was led by Liang Wu of the School of Arts & Sciences and conducted by Yishuai Xu, a postdoc in Wu's lab, and graduate students Zhuoliang Ni and Qinwen Deng, in collaboration with researchers from the Weizmann Institute of Science and University of California, Santa Barbara.
Since their discovery, superconductors with the chemical formula AV3Sb5—where A refers to cesium, rubidium, or potassium—have generated immense interest for their exotic properties. The compounds feature a kagome lattice, an unusual atomic arrangement that resembles and takes its name from a Japanese basket-weave pattern of interlaced, corner-sharing triangles. Kagome lattice materials have fascinated researchers for decades because they provide a window into quantum phenomena such as geometrical frustration, topology, and strong correlations.
While previous research on AV3Sb5 has discovered the coexistence of two different cooperative electronic states—the charge-density wave order and superconductivity—the nature of the symmetry breaking that accompanies these states has been unclear. In physics, symmetry refers to a physical or mathematical feature of a system that remains unchanged under certain transformations. When a material transitions from a normal, high-temperature state to an exotic, low-temperature state like superconductivity, it undergoes symmetry breaking. Wu, whose lab develops and uses time-resolved and nonlinear optical techniques to study quantum materials, set out to clarify the nature of symmetry-breaking when AV3Sb5 enters the charge-density wave phase.
AV3Sb5 exhibits what researchers call a "cascade" of symmetry-broken phases. In other words, as the system cools down, it begins to enter a symmetry breaking state, with lower and lower temperatures leading to additional broken symmetries. "In order to use superconductors for applications, we need to understand them," Wu says. "Because superconductivity develops at even lower temperatures, we need to understand the charge-density wave phase first."
In its normal state, AV3Sb5 consists of a hexagonal crystal structure, composed of kagome lattices of vanadium (V) atoms coordinated by antimony (Sb) stacked on top of one another, with sheets of cesium, rubidium, or potassium in between each V-Sb layer. The structure is six-fold rotationally symmetric; when rotated by 60 degrees, it stays the same.
To find out whether AV3Sb5 retains its six-fold symmetry in the charge-density wave phase, the researchers performed scanning birefringence measurements on all three members of the AV3Sb5 family. Birefringence, or double refraction, refers to an optical property exhibited by materials with crystallographically distinct axes, a principal axis and a non-equivalent axis. When light enters the material along the non-equivalent axis, it splits in two, with each ray polarized and traveling at different speeds.
"In a kagome plane, the linear optical response should be the same along any direction, but they're not in AV3Sb5 because between the two kagome layers there's a relative shift," Wu says, explaining that the birefringence measurements revealed the difference between two orthogonal directions in the plane and a phase shift between the two layers that reduces the six-fold rotational symmetry of the materials to two-fold when they enter the charge-density wave state. "This was not clear to the physics community before."
Distinct axes are not the only explanation for the rotation of the light polarization plane. When linearly polarized light encounters a magnetic surface, it also changes, a phenomenon known as the magneto-optical Kerr effect. After separating out the property of birefringence by sending light along the principal axis in samples of AV3Sb5, the researchers used a second optical technique to measure the onset of the Kerr effect. For all three metals, the experiments reveal that the Kerr effect begins in the charge-density wave state. This finding indicates that the formation of charge-density waves breaks another symmetry, time-reversal symmetry. The simplest way to break time-reversal symmetry—which holds that the laws of physics remain the same whether time runs forward or backwards—is to use a permanent magnet, like those we put on a refrigerator, Wu says.
However, the Kerr effect is only observable at low temperatures with high resolution, indicating that the kagome metals are not substantially magnetic. "With these quantum materials," Wu says, he and his collaborators theorize that time-reversal symmetry is "not broken by a permanent magnet but by a circulating loop current." To confirm the nature of time-reversal symmetry breaking in the charge-density wave state, the researchers performed a third experiment in which they measured the circular dichroism, or the unequal reflectivity of left-handed and right-handed circularly polarized light, of the charge density wave phase. "We still need further work, but this finding really supports the possibility of circulating loop currents," the existence of which would suggest the unconventional nature of superconductivity in the metals, says Wu.
In 2018 Congress passed the National Quantum Initiative Act, with the goal of advancing research on quantum materials and the development of quantum technology. Quantum materials include those with topological properties and those with correlation, like the kagome metals AV3Sb5. While Wu's previous research centered on the former category and antiferromagnets, he says that the scanning optics technique that he'd developed for these studies presented a "ready and versatile tool" for studying symmetry breaking in new kagome metals.
More at link: https://phys.org/news/2022-11-superconductivity-newly-discovered-kagome-metals.html
Paper:
https://arxiv.org/abs/2109.10809v1
Kagome superconductors AV3Sb5 (A=K, Rb, Cs)
Kun Jiang, Tao Wu, Jia-Xin Yin, Zhenyu Wang, M. Zahid Hasan, Stephen D. Wilson, Xianhui Chen, Jiangping Hu
The quasi two-dimensional (quasi-2D) kagome materials AV3Sb5 (A=K, Rb, Cs) were found to be a prime example of kagome superconductors, a new quantum platform to investigate the interplay between electron correlation effects, topology and geometric frustration. In this review, we report recent progress on the experimental and theoretical studies of AV3Sb5 and provide a broad picture of this fast-developing field in order to stimulate an expanded search for unconventional kagome superconductors. We review the electronic properties of AV3Sb5, the experimental measurements of the charge density wave state, evidence of time-reversal symmetry breaking, and other potential hidden symmetry breaking in these materials. A variety of theoretical proposals and models that address the nature of the time-reversal symmetry breaking are discussed. Finally, we review the superconducting properties of AV3Sb5, especially the potential pairing symmetries and the interplay between superconductivity and the charge density wave state.
Comments: A review on kagome superconductors AV3Sb5, including 14 pages and 9 figures
Subjects: Superconductivity (cond-mat.supr-con); Materials Science (cond-mat.mtrl-sci); Strongly Correlated Electrons (cond-mat.str-el)
Cite as: arXiv:2109.10809 [cond-mat.supr-con]
(or arXiv:2109.10809v1 [cond-mat.supr-con] for this version)
https://doi.org/10.48550/arXiv.2109.10809
Related DOI:
https://doi.org/10.1093/nsr/nwac199
Submission history
From: Kun Jiang [view email]
[v1] Wed, 22 Sep 2021 16:01:25 UTC (8,644 KB)

Last edited by Chromium6 on Tue Dec 27, 2022 1:42 am; edited 1 time in total
Chromium6- Posts : 729
Join date : 2019-11-29
Re: Partial List of Superconductors to Build Out
Interesting that a lot of Superconductors with recent research happen with atoms that are full-stack "red" with single discs and arms "blue" or singles per Mathis:
-------
Scientists Uncover Surprising New Clues to Exotic Superconductors’ Superpowers
By Theresa Duque
March 24, 2022
A research team has uncovered new clues into the exotic behavior of unconventional superconductors – devices that efficiently carry electrical current with zero resistance in ways that defy our previous understanding of physics.
“The hope is that our work may lead to a better understanding of superconductivity, which could find applications in next-gen energy storage, supercomputing, and magnetic levitation trains,” said first author Nikola Maksimovic, a graduate student researcher in Berkeley Lab’s Materials Sciences Division and UC Berkeley’s Physics Department.
The work could also help researchers design more powerful superconducting materials by tuning their chemical makeup at the atomic level. The team, led by Lawrence Berkeley National Laboratory (Berkeley Lab) in collaboration with UC Berkeley, reported their findings in the journal Science.
Conventional superconducting materials like lead or tin become superconducting at temperatures close to zero on the Kelvin scale, or minus 523.4 degrees Fahrenheit. But some unconventional superconductors like cuprates, a type of ceramic metal containing copper and oxygen, somehow become superconducting at relatively high temperatures near or above 100 Kelvin (minus 280 degrees Fahrenheit).
For decades, researchers have struggled to understand how superconducting cuprates work, in part because cuprates are difficult to grow without defects. What’s more is their powerful superconductivity is challenging to switch off – like a race car that keeps on going, even when it’s in neutral. Scientists therefore need a tool to help them understand how superconductivity evolves from different phases at the atomic level, and which formulations have the most potential for real-world applications.
So for the current study, a research team led by James Analytis focused on a material made of cerium-cobalt-indium5 (CeCoIn5) that could mimic a cuprate system. Analytis is a faculty scientist and co-investigator in the Quantum Materials program in Berkeley Lab’s Materials Sciences Division, which provided the funding for this work. He is also a physics professor at UC Berkeley.
To some, CeCoIn5 might seem like an unlikely model to study superconducting cuprates. CeCoIn5 contains neither copper nor oxygen, after all. But despite their differences, cuprates and CeCoIn5 share some key traits: They are both unconventional superconductors with electron density or “spatial symmetry” patterns resembling a four-leaf clover. Such spatial symmetry is like a map highlighting which parts of the superconductor are most densely populated by electrons.
The team also knew from other studies that the superconducting state in CeCoIn5 could be switched on and off with powerful magnets that are currently available in the laboratory, whereas the requisite magnetic fields needed to modulate cuprates far exceed those of even the most sophisticated techniques.
Turning off the superconducting state in CeCoIn5, the team reasoned, would allow them to “look under the hood,” and study how the material’s electrons behave in a normal, non-superconducting state. Since cuprates and CeCoIn5 share similar electronic density patterns, the team inferred that studying CeCoIn5 in all its different phases could provide important new clues into the origins of cuprates’ superconducting capabilities.
“CeCoIn5 is a very useful model system. It’s an unconventional superconductor whose properties are very accessible to experimental techniques at high magnetic fields, some of which are not possible in cuprates,” said first author Nikola Maksimovic, a graduate student researcher in Berkeley Lab’s Materials Sciences Division and the Analytis lab in UC Berkeley’s Physics Department.
To begin testing the material as a potential cuprate model, the researchers grew more than a dozen single-crystals of CeCoIn5 at their Materials Sciences Division lab, and then fabricated experimental devices from those crystals at the Molecular Foundry’s National Center for Electron Microscopy facility.
They tuned some of the CeCoIn5 crystals to the magnetic state by replacing a few indium atoms with cadmium, and tuned other samples to the superconducting state by replacing indium with tin.
Maksimovic measured the electron density of these materials at the National High Magnetic Field Laboratory’s Pulsed Field Facility at Los Alamos National Laboratory using magnetic fields of up to 75 tesla, which is about 1.5 million times stronger than the Earth’s magnetic field.
Then, a team led by Alessandra Lanzara used spectroscopic techniques at Berkeley Lab’s Advanced Light Source to image the CeCoIn5 crystals’ electronic energy structure and superconductivity as a function of chemical composition. Lanzara is a senior faculty scientist and co-investigator in the Quantum Materials program in Berkeley Lab’s Materials Sciences Division and a UC Berkeley physics professor.
Much to their surprise, the researchers found that in chemical compositions where the superconductivity is strongest, the number of free electrons jumps from a small value to a large value, signifying that the material is at a transition point. (A free electron is an electron that is not permanently bound to an atom.) The researchers attributed this transition to the behavior of electrons associated with the cerium atoms.
“There are only a few materials where such a transition is suspected to occur. We have some of the clearest evidence that it actually does, and that’s pretty exciting,” Maksimovic said.
In future studies, the researchers plan to investigate how the transition in CeCoIn5 applies to other unconventional superconductors like cuprates. They also plan to investigate how the transition in CeCoIn5 may affect other physical properties of the material such as thermal conductivity.
More at link: https://newscenter.lbl.gov/2022/03/24/exotic-superconductors-superpowers/

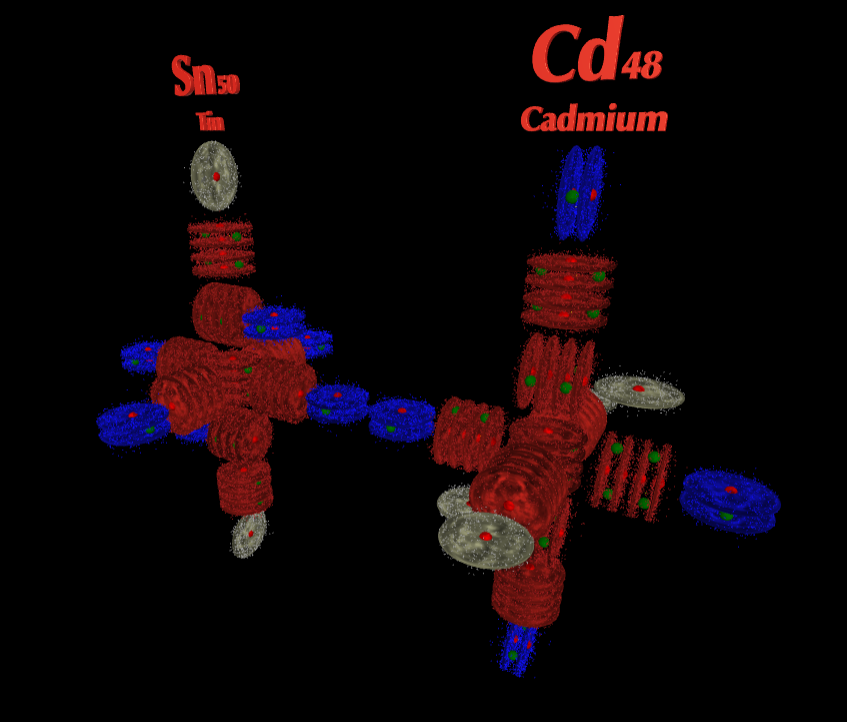
-------
Scientists Uncover Surprising New Clues to Exotic Superconductors’ Superpowers
By Theresa Duque
March 24, 2022
A research team has uncovered new clues into the exotic behavior of unconventional superconductors – devices that efficiently carry electrical current with zero resistance in ways that defy our previous understanding of physics.
“The hope is that our work may lead to a better understanding of superconductivity, which could find applications in next-gen energy storage, supercomputing, and magnetic levitation trains,” said first author Nikola Maksimovic, a graduate student researcher in Berkeley Lab’s Materials Sciences Division and UC Berkeley’s Physics Department.
The work could also help researchers design more powerful superconducting materials by tuning their chemical makeup at the atomic level. The team, led by Lawrence Berkeley National Laboratory (Berkeley Lab) in collaboration with UC Berkeley, reported their findings in the journal Science.
Conventional superconducting materials like lead or tin become superconducting at temperatures close to zero on the Kelvin scale, or minus 523.4 degrees Fahrenheit. But some unconventional superconductors like cuprates, a type of ceramic metal containing copper and oxygen, somehow become superconducting at relatively high temperatures near or above 100 Kelvin (minus 280 degrees Fahrenheit).
For decades, researchers have struggled to understand how superconducting cuprates work, in part because cuprates are difficult to grow without defects. What’s more is their powerful superconductivity is challenging to switch off – like a race car that keeps on going, even when it’s in neutral. Scientists therefore need a tool to help them understand how superconductivity evolves from different phases at the atomic level, and which formulations have the most potential for real-world applications.
So for the current study, a research team led by James Analytis focused on a material made of cerium-cobalt-indium5 (CeCoIn5) that could mimic a cuprate system. Analytis is a faculty scientist and co-investigator in the Quantum Materials program in Berkeley Lab’s Materials Sciences Division, which provided the funding for this work. He is also a physics professor at UC Berkeley.
To some, CeCoIn5 might seem like an unlikely model to study superconducting cuprates. CeCoIn5 contains neither copper nor oxygen, after all. But despite their differences, cuprates and CeCoIn5 share some key traits: They are both unconventional superconductors with electron density or “spatial symmetry” patterns resembling a four-leaf clover. Such spatial symmetry is like a map highlighting which parts of the superconductor are most densely populated by electrons.
The team also knew from other studies that the superconducting state in CeCoIn5 could be switched on and off with powerful magnets that are currently available in the laboratory, whereas the requisite magnetic fields needed to modulate cuprates far exceed those of even the most sophisticated techniques.
Turning off the superconducting state in CeCoIn5, the team reasoned, would allow them to “look under the hood,” and study how the material’s electrons behave in a normal, non-superconducting state. Since cuprates and CeCoIn5 share similar electronic density patterns, the team inferred that studying CeCoIn5 in all its different phases could provide important new clues into the origins of cuprates’ superconducting capabilities.
“CeCoIn5 is a very useful model system. It’s an unconventional superconductor whose properties are very accessible to experimental techniques at high magnetic fields, some of which are not possible in cuprates,” said first author Nikola Maksimovic, a graduate student researcher in Berkeley Lab’s Materials Sciences Division and the Analytis lab in UC Berkeley’s Physics Department.
To begin testing the material as a potential cuprate model, the researchers grew more than a dozen single-crystals of CeCoIn5 at their Materials Sciences Division lab, and then fabricated experimental devices from those crystals at the Molecular Foundry’s National Center for Electron Microscopy facility.
They tuned some of the CeCoIn5 crystals to the magnetic state by replacing a few indium atoms with cadmium, and tuned other samples to the superconducting state by replacing indium with tin.
Maksimovic measured the electron density of these materials at the National High Magnetic Field Laboratory’s Pulsed Field Facility at Los Alamos National Laboratory using magnetic fields of up to 75 tesla, which is about 1.5 million times stronger than the Earth’s magnetic field.
Then, a team led by Alessandra Lanzara used spectroscopic techniques at Berkeley Lab’s Advanced Light Source to image the CeCoIn5 crystals’ electronic energy structure and superconductivity as a function of chemical composition. Lanzara is a senior faculty scientist and co-investigator in the Quantum Materials program in Berkeley Lab’s Materials Sciences Division and a UC Berkeley physics professor.
Much to their surprise, the researchers found that in chemical compositions where the superconductivity is strongest, the number of free electrons jumps from a small value to a large value, signifying that the material is at a transition point. (A free electron is an electron that is not permanently bound to an atom.) The researchers attributed this transition to the behavior of electrons associated with the cerium atoms.
“There are only a few materials where such a transition is suspected to occur. We have some of the clearest evidence that it actually does, and that’s pretty exciting,” Maksimovic said.
In future studies, the researchers plan to investigate how the transition in CeCoIn5 applies to other unconventional superconductors like cuprates. They also plan to investigate how the transition in CeCoIn5 may affect other physical properties of the material such as thermal conductivity.
More at link: https://newscenter.lbl.gov/2022/03/24/exotic-superconductors-superpowers/

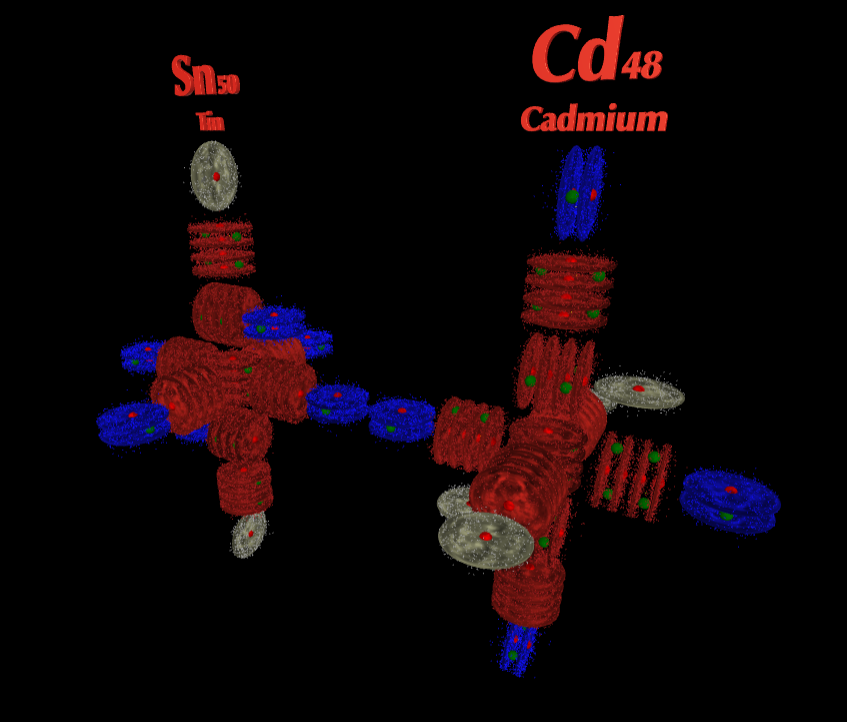
Last edited by Chromium6 on Tue Dec 27, 2022 2:22 am; edited 1 time in total
Chromium6- Posts : 729
Join date : 2019-11-29
Re: Partial List of Superconductors to Build Out
Reconciling SuperExchange with Planar Weight Disparity
- Two Sides of the Same Coin -
22 December 2022
E. Joe Eck
Superconductors.ORG (more at link)
Recently the JC Davis Group claimed to have confirmed the electron-coupling mechanism that facilitates high temperature superconductivity (HTSC) in the copper-oxides[1]. They assert that antiferromagnetic spin–spin interactions of electrons, in a process called “superexchange”, are the glue behind Cooper-pairing. Antiferromagnetism is a form of magnetic ordering where electrons align in a regular pattern of neighboring spins rotating in opposite directions. Although the net magnetic field is zero, such a material does have a microscopic magnetic moment at the quantum level. And with magnetization comes inductance. (Hold that thought.)
In 2013 Superconductors.ORG (SCO) discovered that there absolutely had to be a difference in the atomic weight of atoms positioned on opposite sides of oxygen within a crystal lattice for that material to become superconductive. This arrangement was dubbed "planar-weight-disparity"(PWD). Oscillations in this Sr-O-Ca-O configuration are powered by lattice vibrations - the same thermal energy that causes resistance to appear in a normal conductor. Since heavy atoms vibrate slower than light atoms, PWD results in heterodyne compression of the interposed oxygen atoms along the C axis. [See the above graphic where this is depicted in the simplest HTSC structure: the infinite layer.] The oxygen then responds laterally along the A-B axis to affect the interatomic CuO2 "gap". This is the critical timing mechanism that administrates thermal energy within a superconductor. Without periodic compression (modulation) of the oxygen atoms in HTSC cuprates, superconductivity could not be established.
Then in 2014 further research by SCO found a direct correlation between the relative permittivity (dielectric constant) of sub-structures within the superconductor lattice and transition temperature (Tc) (see graphic above). This means that electrostatic charges are at work in the electron-pairing process. And with electrostatic charges comes capacitance. When both inductance and capacitance are powered by thermal energy, a superconductor becomes a resonating electronic circuit. To illustrate this an equivalent electronic schematic has been placed beside an infinite layer structure at page top. Each component's function is noted and the arrow in the middle indicates a variable flow of electrons in sync with the PWD periodic compression rate.
As part of their pairing mechanism discovery the Davis Group also observed changes in the charge transfer gap (CTG) between the copper and oxygen atoms. As the gap narrowed they found the exchange of electrons increased. So, how would PWD affect the charge transfer gap? Jeff Tallon, a recognized authority in the field of superconductivity at Victoria University in New Zealand, speculated: "I would imagine that planar weight disparity would lead to an alternating modulation of the CTG – something like what Seamus Davis showed in his STM measurements. So a modulation rather than an overall shift."(see graphic above right)
So, does this "modulation" of the charge transfer gap by planar weight disparity affect bulk superconductivity? BSCCO's 2212 structure is actually much larger than the infinite layer structure used as an example earlier in this page. The above graphic shows more oxygen atoms are being compressed. Sr is in opposition to both Ca and Bi. So, if anything, the effect of PWD should be more pronounced. To quote the Davis Group's article in PNAS: "X-ray scattering studies of the Bi2Sr2CaCu2O8+x crystal supermodulation demonstrate that ... the displacement amplitude of the C axis supermodulation is greater in the CuO2 layer than in the adjacent SrO layer." This confirms that while superexchange is facilitating superconductivity in BSCCO, PWD is modulating the CuO2 layers and providing an electronic resonance assist. Superexchange and PWD appear to be two sides of the same coin. To read more about superconductivity and resonant electronic circuits click here.
E. Joe Eck
2022, 2023 Superconductors.ORG
All rights reserved.
1. Shane M. O’Mahony, Wangping Ren, Weijiong Chen, Yi Xue Chong, Xiaolong Liu, H. Eisaki, S. Uchida, M. H. Hamidian, and J. C. Séamus Davis

- Two Sides of the Same Coin -
22 December 2022
E. Joe Eck
Superconductors.ORG (more at link)
Recently the JC Davis Group claimed to have confirmed the electron-coupling mechanism that facilitates high temperature superconductivity (HTSC) in the copper-oxides[1]. They assert that antiferromagnetic spin–spin interactions of electrons, in a process called “superexchange”, are the glue behind Cooper-pairing. Antiferromagnetism is a form of magnetic ordering where electrons align in a regular pattern of neighboring spins rotating in opposite directions. Although the net magnetic field is zero, such a material does have a microscopic magnetic moment at the quantum level. And with magnetization comes inductance. (Hold that thought.)
In 2013 Superconductors.ORG (SCO) discovered that there absolutely had to be a difference in the atomic weight of atoms positioned on opposite sides of oxygen within a crystal lattice for that material to become superconductive. This arrangement was dubbed "planar-weight-disparity"(PWD). Oscillations in this Sr-O-Ca-O configuration are powered by lattice vibrations - the same thermal energy that causes resistance to appear in a normal conductor. Since heavy atoms vibrate slower than light atoms, PWD results in heterodyne compression of the interposed oxygen atoms along the C axis. [See the above graphic where this is depicted in the simplest HTSC structure: the infinite layer.] The oxygen then responds laterally along the A-B axis to affect the interatomic CuO2 "gap". This is the critical timing mechanism that administrates thermal energy within a superconductor. Without periodic compression (modulation) of the oxygen atoms in HTSC cuprates, superconductivity could not be established.
Then in 2014 further research by SCO found a direct correlation between the relative permittivity (dielectric constant) of sub-structures within the superconductor lattice and transition temperature (Tc) (see graphic above). This means that electrostatic charges are at work in the electron-pairing process. And with electrostatic charges comes capacitance. When both inductance and capacitance are powered by thermal energy, a superconductor becomes a resonating electronic circuit. To illustrate this an equivalent electronic schematic has been placed beside an infinite layer structure at page top. Each component's function is noted and the arrow in the middle indicates a variable flow of electrons in sync with the PWD periodic compression rate.
As part of their pairing mechanism discovery the Davis Group also observed changes in the charge transfer gap (CTG) between the copper and oxygen atoms. As the gap narrowed they found the exchange of electrons increased. So, how would PWD affect the charge transfer gap? Jeff Tallon, a recognized authority in the field of superconductivity at Victoria University in New Zealand, speculated: "I would imagine that planar weight disparity would lead to an alternating modulation of the CTG – something like what Seamus Davis showed in his STM measurements. So a modulation rather than an overall shift."(see graphic above right)
So, does this "modulation" of the charge transfer gap by planar weight disparity affect bulk superconductivity? BSCCO's 2212 structure is actually much larger than the infinite layer structure used as an example earlier in this page. The above graphic shows more oxygen atoms are being compressed. Sr is in opposition to both Ca and Bi. So, if anything, the effect of PWD should be more pronounced. To quote the Davis Group's article in PNAS: "X-ray scattering studies of the Bi2Sr2CaCu2O8+x crystal supermodulation demonstrate that ... the displacement amplitude of the C axis supermodulation is greater in the CuO2 layer than in the adjacent SrO layer." This confirms that while superexchange is facilitating superconductivity in BSCCO, PWD is modulating the CuO2 layers and providing an electronic resonance assist. Superexchange and PWD appear to be two sides of the same coin. To read more about superconductivity and resonant electronic circuits click here.
E. Joe Eck

All rights reserved.
1. Shane M. O’Mahony, Wangping Ren, Weijiong Chen, Yi Xue Chong, Xiaolong Liu, H. Eisaki, S. Uchida, M. H. Hamidian, and J. C. Séamus Davis

Chromium6- Posts : 729
Join date : 2019-11-29
Re: Partial List of Superconductors to Build Out
Found this site which has recent papers on what people are looking globally for super conductors and other structures. Found more datasets-papers here for formulas. Has data files with the Formulas researched with some papers:
https://archive.materialscloud.org/
A recent graphene paper:
https://archive.materialscloud.org/record/2023.60
Ab initio real-time quantum dynamics of charge carriers in momentum space
Zhenfa Zheng1, Yongliang Shi1,2,3*, Jin-Jian Zhou4, Oleg V. Prezhdo5, Qijing Zheng1*, Jin Zhao1,6*
1 Department of Physics, ICQD/Hefei National Research Center for Physical Sciences at Microscale, University of Science and Technology of China, Hefei, 230026, Anhui, China
2 Center for Spintonics and Quantum Systerms, State Key Laboratory for Mechanical Behavior of Materials, School of Materials Science and Engineering, Xi’an Jiaotong University, Xi’an, 710049, Shanxi, China
3 State Key Laboratory of Surface Physics and Department of Physics, Fudan University, Shanghai, 200433, China
4 School of Physics, Beijing Institute of Technology, Beijing, 100081, China
5 Departments of Chemistry, Physics, and Astronomy, University of Southern California, Los Angeles, 90089, California, USA
6 Department of Physics and Astronomy, University of Pittsburgh, Pittsburgh, 15260, Pennsylvania, USA
* Corresponding authors emails: sylcliff@xjtu.edu.cn, zgj@ustc.edu.cn, zhaojin@ustc.edu.cn
DOI10.24435/materialscloud:2n-3j [version v3]
Publication date: Apr 12, 2023
How to cite this record
Zhenfa Zheng, Yongliang Shi, Jin-Jian Zhou, Oleg V. Prezhdo, Qijing Zheng, Jin Zhao, Ab initio real-time quantum dynamics of charge carriers in momentum space, Materials Cloud Archive 2023.60 (2023), doi: 10.24435/materialscloud:2n-3j.
Description
Application of the nonadiabatic molecular dynamics (NAMD) approach is severely limited to studying carrier dynamics in the momentum space, since a supercell is required to sample the phonon excitation and electron-phonon (e-ph) interaction at different momenta in a molecular dynamics simulation. Here, we develop an ab initio approach for the real-time quantum dynamics for charge carriers in the momentum space (NAMD_k) by directly introducing the e-ph coupling into the Hamiltonian based on the harmonic approximation. The NAMD_k approach maintains the quantum zero-point energy and proper phonon dispersion, and includes memory effects of phonon excitation. The application of NAMD_k to the hot carrier dynamics in graphene reveals the phonon-specific relaxation mechanism. An energy threshold of 0.2eV, defined by two optical phonon modes strongly coupled to the electrons, separates the hot electron relaxation into fast and slow regions with the lifetimes of pico- and nano-seconds, respectively. The NAMD_k approach provides a powerful tool to understand real-time carrier dynamics in the momentum space for different materials.
Materials Cloud sections using this data
https://archive.materialscloud.org/
A recent graphene paper:
https://archive.materialscloud.org/record/2023.60
Ab initio real-time quantum dynamics of charge carriers in momentum space
Zhenfa Zheng1, Yongliang Shi1,2,3*, Jin-Jian Zhou4, Oleg V. Prezhdo5, Qijing Zheng1*, Jin Zhao1,6*
1 Department of Physics, ICQD/Hefei National Research Center for Physical Sciences at Microscale, University of Science and Technology of China, Hefei, 230026, Anhui, China
2 Center for Spintonics and Quantum Systerms, State Key Laboratory for Mechanical Behavior of Materials, School of Materials Science and Engineering, Xi’an Jiaotong University, Xi’an, 710049, Shanxi, China
3 State Key Laboratory of Surface Physics and Department of Physics, Fudan University, Shanghai, 200433, China
4 School of Physics, Beijing Institute of Technology, Beijing, 100081, China
5 Departments of Chemistry, Physics, and Astronomy, University of Southern California, Los Angeles, 90089, California, USA
6 Department of Physics and Astronomy, University of Pittsburgh, Pittsburgh, 15260, Pennsylvania, USA
* Corresponding authors emails: sylcliff@xjtu.edu.cn, zgj@ustc.edu.cn, zhaojin@ustc.edu.cn
DOI10.24435/materialscloud:2n-3j [version v3]
Publication date: Apr 12, 2023
How to cite this record
Zhenfa Zheng, Yongliang Shi, Jin-Jian Zhou, Oleg V. Prezhdo, Qijing Zheng, Jin Zhao, Ab initio real-time quantum dynamics of charge carriers in momentum space, Materials Cloud Archive 2023.60 (2023), doi: 10.24435/materialscloud:2n-3j.
Description
Application of the nonadiabatic molecular dynamics (NAMD) approach is severely limited to studying carrier dynamics in the momentum space, since a supercell is required to sample the phonon excitation and electron-phonon (e-ph) interaction at different momenta in a molecular dynamics simulation. Here, we develop an ab initio approach for the real-time quantum dynamics for charge carriers in the momentum space (NAMD_k) by directly introducing the e-ph coupling into the Hamiltonian based on the harmonic approximation. The NAMD_k approach maintains the quantum zero-point energy and proper phonon dispersion, and includes memory effects of phonon excitation. The application of NAMD_k to the hot carrier dynamics in graphene reveals the phonon-specific relaxation mechanism. An energy threshold of 0.2eV, defined by two optical phonon modes strongly coupled to the electrons, separates the hot electron relaxation into fast and slow regions with the lifetimes of pico- and nano-seconds, respectively. The NAMD_k approach provides a powerful tool to understand real-time carrier dynamics in the momentum space for different materials.
Materials Cloud sections using this data
Chromium6- Posts : 729
Join date : 2019-11-29
Re: Partial List of Superconductors to Build Out
Sounds like more Charge Field description per Miles.
----------
DECEMBER 15, 2023
Editors' notes
Ultrafast lasers map electrons 'going ballistic' in graphene with implications for next-gen electronic devices
by Brendan M. Lynch, University of Kansas
Research at the University of Kansas' Ultrafast Laser Lab could lead to breakthroughs in governing electrons in semiconductors, fundamental components in most information and energy technology. Credit: University of Kansas
Research appearing in ACS Nano reveals the ballistic movement of electrons in graphene in real-time.
The observations made at the University of Kansas' Ultrafast Laser Lab could lead to breakthroughs in governing electrons in semiconductors, fundamental components in most information and energy technology.
"Generally, electron movement is interrupted by collisions with other particles in solids," said lead author Ryan Scott, a doctoral student in KU's Department of Physics & Astronomy.
"This is similar to someone running in a ballroom full of dancers. These collisions are rather frequent—about 10 to 100 billion times per second. They slow down the electrons, cause energy loss, and generate unwanted heat. Without collisions, an electron would move uninterrupted within a solid, similar to cars on a freeway or ballistic missiles through the air. We refer to this as 'ballistic transport.'"
Scott performed the lab experiments under the mentorship of Hui Zhao, a professor of physics & astronomy at KU. They were joined in the work by former KU doctoral student Pavel Valencia-Acuna, now a postdoctoral researcher at the Northwest Pacific National Laboratory.
Zhao said electronic devices utilizing ballistic transport could potentially be faster, more powerful, and more energy efficient.
"Current electronic devices, such as computers and phones, utilize silicon-based field-effect transistors," Zhao said. "In such devices, electrons can only drift with a speed on the order of centimeters per second due to the frequent collisions they encounter. The ballistic transport of electrons in graphene can be utilized in devices with fast speed and low energy consumption."
The KU researchers observed the ballistic movement in graphene, a promising material for next-generation electronic devices. First discovered in 2004 and awarded the Nobel Prize in Physics in 2010, graphene is made of a single layer of carbon atoms forming a hexagonal lattice structure—somewhat like a soccer net.
"Electrons in graphene move as if their 'effective' mass is zero, making them more likely to avoid collisions and move ballistically," Scott said. "Previous electrical experiments, by studying electrical currents produced by voltages under various conditions, have revealed signs of ballistic transport. However, these techniques aren't fast enough to trace the electrons as they move."
According to the researchers, electrons in graphene (or any other semiconductor) are like students sitting in a full classroom, where students can't freely move around because the desks are full. The laser light can free electrons to momentarily vacate a desk, or 'hole' as physicists call them.
"Light can provide energy to an electron to liberate it so that it can move freely," Zhao said. "This is similar to allowing a student to stand up and walk away from their seat. However, unlike a charge-neutral student, an electron is negatively charged. Once the electron has left its 'seat," the seat becomes positively charged and quickly drags the electron back, resulting in no more mobile electrons—like the student sitting back down."
Because of this effect, the super-light electrons in graphene can only stay mobile for about one trillionth of a second before falling back to its seat. This short time presents a severe challenge to observing the movement of the electrons. To address this problem, the KU researchers designed and fabricated a four-layer artificial structure with two graphene layers separated by two other single-layer materials, molybdenum disulfide, and molybdenum diselenide.
"With this strategy, we were able to guide the electrons to one graphene layer while keeping their 'seats' in the other graphene layer," Scott said. "Separating them with two layers of molecules, with a total thickness of just 1.5 nanometers, forces the electrons to stay mobile for about 50-trillionths of a second, long enough for the researchers, equipped with lasers as fast as 0.1 trillionths of a second, to study how they move."
The researchers use a tightly focused laser spot to liberate some electrons in their sample. They trace these electrons by mapping out the "reflectance" of the sample, or the percentage of light they reflect.
"We see most objects because they reflect light to our eyes," Scott said.
"Brighter objects have larger reflectance. On the other hand, dark objects absorb light, which is why dark clothes become hot in the summer. When a mobile electron moves to a certain location of the sample, it makes that location slightly brighter by changing how electrons in that location interact with light. The effect is very small—even with everything optimized, one electron only changes the reflectance by 0.1 part per million."
To detect such a small change, the researchers liberated 20,000 electrons at once, using a probe laser to reflect off the sample and measure this reflectance, repeating the process 80 million times for each data point. They found the electrons, on average, move ballistically for about 20 trillionths of a second with a speed of 22 kilometers per second before running into something that terminates their ballistic motion.
More information: Ryan J. Scott et al, Spatiotemporal Observation of Quasi-Ballistic Transport of Electrons in Graphene, ACS Nano (2023). DOI: 10.1021/acsnano.3c08816
https://phys.org/news/2023-12-ultrafast-lasers-electrons-ballistic-graphene.html
----------
DECEMBER 15, 2023
Editors' notes
Ultrafast lasers map electrons 'going ballistic' in graphene with implications for next-gen electronic devices
by Brendan M. Lynch, University of Kansas
Research at the University of Kansas' Ultrafast Laser Lab could lead to breakthroughs in governing electrons in semiconductors, fundamental components in most information and energy technology. Credit: University of Kansas
Research appearing in ACS Nano reveals the ballistic movement of electrons in graphene in real-time.
The observations made at the University of Kansas' Ultrafast Laser Lab could lead to breakthroughs in governing electrons in semiconductors, fundamental components in most information and energy technology.
"Generally, electron movement is interrupted by collisions with other particles in solids," said lead author Ryan Scott, a doctoral student in KU's Department of Physics & Astronomy.
"This is similar to someone running in a ballroom full of dancers. These collisions are rather frequent—about 10 to 100 billion times per second. They slow down the electrons, cause energy loss, and generate unwanted heat. Without collisions, an electron would move uninterrupted within a solid, similar to cars on a freeway or ballistic missiles through the air. We refer to this as 'ballistic transport.'"
Scott performed the lab experiments under the mentorship of Hui Zhao, a professor of physics & astronomy at KU. They were joined in the work by former KU doctoral student Pavel Valencia-Acuna, now a postdoctoral researcher at the Northwest Pacific National Laboratory.
Zhao said electronic devices utilizing ballistic transport could potentially be faster, more powerful, and more energy efficient.
"Current electronic devices, such as computers and phones, utilize silicon-based field-effect transistors," Zhao said. "In such devices, electrons can only drift with a speed on the order of centimeters per second due to the frequent collisions they encounter. The ballistic transport of electrons in graphene can be utilized in devices with fast speed and low energy consumption."
The KU researchers observed the ballistic movement in graphene, a promising material for next-generation electronic devices. First discovered in 2004 and awarded the Nobel Prize in Physics in 2010, graphene is made of a single layer of carbon atoms forming a hexagonal lattice structure—somewhat like a soccer net.
"Electrons in graphene move as if their 'effective' mass is zero, making them more likely to avoid collisions and move ballistically," Scott said. "Previous electrical experiments, by studying electrical currents produced by voltages under various conditions, have revealed signs of ballistic transport. However, these techniques aren't fast enough to trace the electrons as they move."
According to the researchers, electrons in graphene (or any other semiconductor) are like students sitting in a full classroom, where students can't freely move around because the desks are full. The laser light can free electrons to momentarily vacate a desk, or 'hole' as physicists call them.
"Light can provide energy to an electron to liberate it so that it can move freely," Zhao said. "This is similar to allowing a student to stand up and walk away from their seat. However, unlike a charge-neutral student, an electron is negatively charged. Once the electron has left its 'seat," the seat becomes positively charged and quickly drags the electron back, resulting in no more mobile electrons—like the student sitting back down."
Because of this effect, the super-light electrons in graphene can only stay mobile for about one trillionth of a second before falling back to its seat. This short time presents a severe challenge to observing the movement of the electrons. To address this problem, the KU researchers designed and fabricated a four-layer artificial structure with two graphene layers separated by two other single-layer materials, molybdenum disulfide, and molybdenum diselenide.
"With this strategy, we were able to guide the electrons to one graphene layer while keeping their 'seats' in the other graphene layer," Scott said. "Separating them with two layers of molecules, with a total thickness of just 1.5 nanometers, forces the electrons to stay mobile for about 50-trillionths of a second, long enough for the researchers, equipped with lasers as fast as 0.1 trillionths of a second, to study how they move."
The researchers use a tightly focused laser spot to liberate some electrons in their sample. They trace these electrons by mapping out the "reflectance" of the sample, or the percentage of light they reflect.
"We see most objects because they reflect light to our eyes," Scott said.
"Brighter objects have larger reflectance. On the other hand, dark objects absorb light, which is why dark clothes become hot in the summer. When a mobile electron moves to a certain location of the sample, it makes that location slightly brighter by changing how electrons in that location interact with light. The effect is very small—even with everything optimized, one electron only changes the reflectance by 0.1 part per million."
To detect such a small change, the researchers liberated 20,000 electrons at once, using a probe laser to reflect off the sample and measure this reflectance, repeating the process 80 million times for each data point. They found the electrons, on average, move ballistically for about 20 trillionths of a second with a speed of 22 kilometers per second before running into something that terminates their ballistic motion.
More information: Ryan J. Scott et al, Spatiotemporal Observation of Quasi-Ballistic Transport of Electrons in Graphene, ACS Nano (2023). DOI: 10.1021/acsnano.3c08816
https://phys.org/news/2023-12-ultrafast-lasers-electrons-ballistic-graphene.html
Chromium6- Posts : 729
Join date : 2019-11-29
Page 2 of 2 • 1, 2

» May have found a partial cure for Type-1 Diabetes thanks to Miles Mathis
» Dendritic flux avalanches in superconductors
» Magnets levitate above a superconductor: New properties of superconductors discovered
» A List of the Units -- the smallest single measures (1=x)
» Dendritic flux avalanches in superconductors
» Magnets levitate above a superconductor: New properties of superconductors discovered
» A List of the Units -- the smallest single measures (1=x)
Page 2 of 2
Permissions in this forum:
You cannot reply to topics in this forum
|
|